wNMR — An Emerging Analytical Technology: From Biomanufacturing to Point-Of-Care
By Yihua Bruce Yu, Ph.D., Katharine T. Briggs, Ph.D., and Marc B. Taraban, Ph.D.
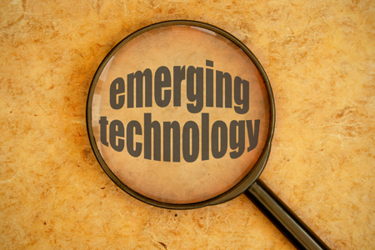
Analytical technologies that are used in the pharmaceutical industry often have their origin in academic research, where a heavy premium is put on thoroughly understanding the structure of a molecule and the mechanistic details of its interactions. Structure and mechanism, not necessarily practicality, reign supreme in academic scientific research, and far less emphasis is put on measuring things as they are in a timely, simple, convenient, and cost-effective manner. But providing a means to measure things as they are may enable data collection in situations where no data existed before, and thus new knowledge and new concepts may emerge. In this article, we present an emerging analytical technology, water proton nuclear magnetic resonance (wNMR), in the context of biologics production and quality assurance, in settings where there is no comparable technology available and, in our view, where it fills a data gap.
The pursuit of structural and mechanistic understanding, especially using biomolecular NMR spectroscopy, has indeed yielded a cornucopia of biomolecular structures and dynamics with ever finer details, which undoubtedly are of tremendous value to both basic biology and drug discovery. But biomolecular NMR spectroscopy work requires highly trained personnel, and NMR sample preparation can be laborious, as biomolecules often require isotopic labeling/enrichment. Data collection may take days if not weeks, and data processing often take days to months. High-field NMR spectroscopy is not well suited for manufacturing plants, clinics, pharmacies, or patient homes.
wNMR is a noninvasive analytical technology that can measure things as they are, in a simple, rapid, and straightforward fashion. Because of its simplicity, wNMR is an analytical tool that can operate in a variety of settings where other analytical technologies are ill suited. We envision that wNMR could be used by workers at pharmaceutical manufacturing plants to monitor the production line, by pharmacists at retail pharmacies to check every vial before dispensing to customers, and eventually by patients before self-injecting biologics for chronic conditions, such as insulin for diabetes management. wNMR can be used in a broad range of situations and, combined with its affordability, we anticipate this technology has the potential for wide adoption.
The concept of an analytical technology for the masses grows out of the realization that for many real-world situations, what is needed is sufficient information for on-the-spot decision-making, such as whether to continue or to stop a production process, to accept or to reject a vaccine vial, to dispense a drug product to a customer or to discard it, and to self-inject or not an insulin pen stored in a cooler after a long drive during summer vacation. What is needed in these situations is quick and reliable detection of an abnormality or change, not the chemical or biological details underlying the abnormality or change; that can come later. wNMR can yield useful information quickly and, with further development, could be used by people with minimal training, similar to people using cell phones after minimal training.
In fact, we see analytical technologies for the masses as analogous to communication technologies for the masses, such as cell phones. Cell phones are mini-computers, and their computational capacity is primitive relative to supercomputers. But the transformative power of cell phones is here for all to see. People use their cell phones to check on the weather, but they do not use their phones to predict the weather. Weather prediction is made by highly trained meteorologists and other specialists at national/regional centers like the NOAA, using complex mathematical models and supercomputers. Similarly, at vaccination sites, nurses could benefit from an analytical technology capable of detecting outlier vials and putting them aside for further investigations to be conducted by highly trained specialists at national/regional centers like the CDC, where more sophisticated analytical technologies can be used.
In our opinion, analytical technologies for the masses like wNMR have the potential to transform the society. By enabling manufacturers and end users of pharmaceuticals to collect data in various real-world settings, new knowledge about pharmaceutical quality may emerge and new practice norms may be established. For example, quality control (QC) for vaccines and other biologics may transition from current inference-based practices, where a few vials in a batch are quantitatively sampled before release, to verification-based practices, where every vial in a batch is quantitatively inspected before release by manufacturers and then before injection by end users.1 Point-of-care QC provides another layer for patient protection.
What Is wNMR And Why Is It Practical For Pharmaceutical Industry Use?
In wNMR, the signal source is the hydrogen nuclei of water, or water protons (1H2O). This is the fundamental difference between wNMR and conventional NMR spectroscopy, where the signal source is the hydrogen or other nuclei of the solutes, such as proteins, nucleic acids, lipids, excipients, adjuvants, etc. The motivation for switching the signal source from the solutes to the solvent in wNMR is the huge NMR signal gain, which compensates for loss in resolution at the lower field-strength compared to conventional NMR. In NMR, the signal intensity is proportional to the concentration of the signal source and the solvent concentration is much higher than that of the solutes. In typical aqueous solutions or suspensions relevant to biologics production and formulation, the water concentration is 103 to 106 higher than that of any solute. The high concentration of water protons, typically in the 80 to 100 M range, makes the detection of the 1H2O signal very simple and fast.
Another important difference between wNMR and conventional NMR spectroscopy is that data analysis of wNMR is performed in the time domain while data analysis of the latter is performed primarily in the frequency domain. In wNMR, the primary experimental parameters are the relaxation rates of water protons, including the longitudinal relaxation rate R1(1H2O) and the transverse relaxation rate R2(1H2O). In conventional NMR, the primary experimental parameters are the chemical shift d and related spectral quantities of the solutes in the frequency domain, although the relaxation rates of various solute nuclei are also used in the study of molecular dynamics.
With water as the signal source, wNMR uses inexpensive and portable benchtop NMR instruments with wide bore size (18 to 50 mm, or higher). A wide bore size is important as it allows a vial/bottle/pen/syringe to be positioned inside the cavity of an NMR probe and, therefore, to be inspected intact. Data collection and processing typically takes seconds, which makes real-time process monitoring and high-throughput product inspection possible.
wNMR truly measures samples as they are. There is no sample preparation of any kind. Data can be collected in a noninvasive, contact-free manner on sealed containers (vials, syringes, pens, etc.) for product inspection (Figure 1) and pipelines/loops for in-line, real-time continuous process monitoring (Figure 2). In this regard, wNMR bears resemblance to medical MRI that noninvasively collects brain images and monitors blood flow, among other things, without any physical contact. This resemblance is not coincidental. In both wNMR and MRI, the signal source is the water protons. Also, water proton relaxation plays important roles in both wNMR and MRI.
Figure 1. A vial of the vaccine Daptacel being lowered into the bore of a benchtop NMR instrument for inspection.
Figure 2. Setup of a flow benchtop NMR instrument, a potential analytical technology for contact-free in-line, real-time process monitoring. The flow direction in the closed loop is indicated with yellow arrows.
Why Is The 1H2O NMR Signal Informative About The Solute?
Water, as the solvent, has extensive interactions with solutes dissolved or suspended in it. Such water-solute interactions make the 1H2O NMR signal sensitive to the physicochemical status and/or structural organization of the solutes. Specific mechanisms may include water-solute proton exchange, magnetic susceptibility contrast between water and solute particles, and compartmentalization of water molecules by solute macromolecules or particles.2
Earlier studies have used the relaxation dynamics of water to probe the mobility of water molecules in protein solutions.3 In wNMR, however, water is used as a spectroscopic probe for solutes, not water itself.
Why Use NMR But Not Raman Or IR?
In addition to the NMR signal, water also emits Raman and IR (infrared) signals. One might ask why not use the water Raman or IR signals to probe the physicochemical status of solutes? The answer is that in order to use property X of the solvent to indirectly probe the solutes, X needs to be sensitive to the solutes via noncovalent interactions. Further, a significant portion of the solvent molecules need to have their property X altered by solvent-solute interactions. To this end, X needs to be of low energy, so it can be easily perturbed by the subtle changes in the properties and/or organization of the solutes, and of long lifetime, so there is sufficient time for solvent molecules with altered X to accumulate (this will not happen if the signal dies out too quickly). NMR is chosen over Raman and IR because its energy (~107 Hz) is low and its lifetime is long (~100 s). In contrast, Raman and IR signals have much higher energies (~1014 Hz) and much shorter lifetimes (~10-12 s). An additional benefit of NMR is that it has no requirement for sample optical transparency at all. Many biologic products such as insulin pens and labeled vaccine vials are entirely nontransparent.
What Can wNMR Do?
Since 2014, we have published 20+ papers on various topics of wNMR, ranging from sensing biomaterial stiffness4 to detecting nanoparticle clustering.2 The abbreviation wNMR was first used to shorten “time-domain water proton NMR” in an article on detecting monoclonal antibody (mAb) aggregation5 and now seems to have been adopted by the broader community.6 Of interest to biologics production and quality control, wNMR can detect protein aggregation under both static5,7 and flow8 conditions, monitor aluminum adjuvant filling level9 and sedimentation kinetics,10 inspect marketed biologic products such as insulins11,12 and vaccines,13,14 and could be used to quantify the gene filling level in viral capsids.15 These studies demonstrate that wNMR may be used in biologics formulation (e.g., excipient screening), production (e.g., in-line process monitoring), and inspection (e.g., quantitative inspection of vials).
An unexpected finding from our studies is vial-to-vial variability of freezing susceptibility among vials of vaccines from the same carton.13,14 Although variability of freezing susceptibility does not necessarily translate to variability of safety or efficacy, this finding demonstrates that vial-to-vial variability does exist and wNMR can detect such variability.
What Can wNMR Not Do?
As a noninvasive analytical technology, wNMR is suited for real-time process monitoring and on-the-spot product inspection. Its main function is to detect an abnormality, such as unexpected process spikes or outlier product vials. wNMR alone cannot specify the chemical and biological details underlying the detected anomaly. When anomalies are detected, they can be examined in greater detail using conventional invasive analytical technologies, such as LC-MS or high-field NMR spectroscopy, among many others.
It is worth noting that wNMR could miss some anomalies. Like all analytical technologies, wNMR has detection limits. For example, wNMR may not be able detect trace contaminants, such as host cell proteins or nucleic acids, microbials, and endotoxins. Also, subtle changes in protein glycosylation may escape detection by wNMR. The exact detection limit for each type of anomaly by wNMR needs to be established in a case-by-case fashion.
It is also possible that some anomalies may cancel each other’s impact on water proton relaxation, resulting in false negatives. Such false negatives may be overcome by expanding wNMR from its simplest univariate form, which uses a single R1(1H2O) or R2(1H2O) for anomaly detection, to more sophisticated multivariate forms, such as R1(1H2O)-R2(1H2O) correlation spectra and interpulse dispersion profiles of R2(1H2O), etc. Such multivariate wNMR data in essence constitute a fingerprint of a process or a product, thereby greatly enhancing its discerning power and making false negatives much less likely.
As a noninvasive analytical technology, wNMR is still at its early stage. Its capabilities and limitations will be revealed through further research and development.
Conclusion
Water, in various forms, appears in almost every aspect of our daily life. wNMR reveals that water can encode a tremendous amount of information. What is needed are technologies to decode the information. When the technologies are made suitable for mass deployment, like cell phones, our lives may be transformed.
References
- Yu YB, Taraban MB, Wang W, Briggs KT. Improving Biopharmaceutical Safety through Verification-Based Quality Control. Trends Biotechnol. 2017;35: 1140–1155. doi:10.1016/j.tibtech.2017.08.010
- Taraban MB, Truong HC, Ilavsky J, DePaz RA, Lobo B, Yu YB. Noninvasive detection of nanoparticle clustering by water proton NMR. Transl Mater Res. 2017;4: 025002. doi:10.1088/2053-1613/aa7838
- K.-Daszkiewicz O, Hennel JW, Lubas B, Szczepkowski TW. Proton magnetic relaxation and protein hydration. Nature. 1963;200: 1006–1007. doi:10.1038/2001006a0
- Feng Y, Taraban MB, Yu YB. Linear dependence of the water proton transverse relaxation rate on the shear modulus of hydrogels. Chem Commun. 2014;50: 12120–12122. doi:10.1039/c4cc04717f
- Taraban MB, DePaz RA, Lobo B, Yu YB. Water Proton NMR: A Tool for Protein Aggregation Characterization. Anal Chem. 2017;89: 5494–5502. doi:10.1021/acs.analchem.7b00464
- Hartl M. wNMR Analytics of Biologics: IPC or Release Analytics Done in Seconds! In: The 13th Annual Bioprocessing Summit [Internet]. 2021 [cited 8 Nov 2021]. Available: https://www.bioprocessingsummit.com/preclinical-analytical-development#MaximilianHartl
- Feng Y, Taraban MB, Yu YB. Water proton NMR-a sensitive probe for solute association. Chem Commun. 2015;51: 6804–6807. doi:10.1039/c5cc00741k
- Taraban MB, Briggs KT, Merkel P, Yu YB. Flow Water Proton NMR: In-Line Process Analytical Technology for Continuous Biomanufacturing. Anal Chem. 2019;91: 13538–13546. doi:10.1021/acs.analchem.9b02622
- Taraban MB, Fox CB, Yu YB. Assessing Aluminum Vaccine Adjuvant Filling, Sedimentation, and Resuspension in Sealed Vials using Water Proton NMR. Am Pharm Rev. 2019;22: 70–73.
- Taraban MB, Yu YB. Monitoring of the sedimentation kinetics of vaccine adjuvants using water proton NMR relaxation. Magn Reson Chem. 2021;59: 147–161. doi:https://doi.org/10.1002/mrc.5096
- Briggs KT, Taraban MB, Wang W, Yu YB. Nondestructive Quantitative Inspection of Drug Products Using Benchtop NMR Relaxometry-the Case of NovoMix® 30. AAPS PharmSciTech. 2019; 214. doi:10.1208/s12249-019-1428-6
- Taraban MB, Wang Y, Briggs KT, Yu YB. Inspecting Insulin Products Using Water Proton NMR. I. Noninvasive vs. Invasive Inspection. J Diabetes Sci Technol. 2021. doi:https://doi.org/10.1177/19322968211023806
- Briggs KT, Taraban MB, Yu YB. Quality assurance at the point-of-care: Noninvasively detecting vaccine freezing variability using water proton NMR. Vaccine. 2020;38: 4853–4860. doi:10.1016/j.vaccine.2020.05.049
- Briggs KT, Taraban MB, Yu YB. Using Water Proton NMR to Characterize Aluminum-adjuvanted Vaccines. In: Mantle M, Hughes L, editors. Magnetic Resonance and its Applications in Drug Formulation and Delivery: New Developments in NMR. MRC Book Series; 2021. Accepted.
- Taraban MB, Jones MT, Yu YB. Rapid and Noninvasive Quantification of Capsid Gene Filling Level Using Water Proton Nuclear Magnetic Resonance. Anal Chem. 2021;93:15816–15820. doi:10.1021/acs.analchem.1c04088
About The Authors:
Y. Bruce Yu, Ph.D., is an inaugural MPower professor at the University of Maryland and the director of its Bio- and Nano-Technology Center. Yu’s expertise is in biophysics and bioengineering. He works at the Institute for Bioscience and Biotechnology Research, a joint research institute between the University of Maryland and the National Institute of Standards and Technology. His lab advances the development of analytical technologies for characterizing complex drugs and vaccines. He received the 2006 U.S. Presidential Early Careers Awards for Scientists and Engineers.
Katharine T. Briggs, Ph.D., is a research associate in the Department of Pharmaceutical Sciences, School of Pharmacy at the University of Maryland (Baltimore) in the lab of Prof. Bruce Yu, where she is exploring time-domain NMR parameters for use in the pharmaceutical industry. Currently, her research evaluates drug products, such as insulin pens and vaccines, using various time-domain water proton NMR methods to non-invasively detect product changes. Prior research includes using high-field NMR to study lithium binding to small molecules to elucidate the mode of lithium binding.
Marc B. Taraban, Ph.D., is an assistant professor (research) in the Department of Pharmaceutical Sciences, School of Pharmacy, University of Maryland (Baltimore). The scope of his research interests and experience spans from chemical physics and biophysics, structural biology, and biomaterials to the applications of dynamic NMR (relaxation) to characterize a variety of pharmaceuticals, including biologics and nanoparticle products.