The Future Of Influenza Vaccines: Self-Amplifying mRNA
By Roberta Duncan, mRNA program lead, Seqirus
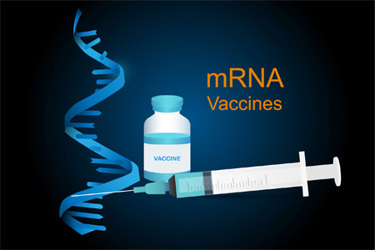
While the first paper to show that messenger RNA (mRNA) could be used as a vaccine was published in 1990, the efficacy of an mRNA vaccine in a Phase 3 clinical trial was not determined until 2020 in the midst of the COVID-19 pandemic.1,2 Given the profound impact of the COVID-19 pandemic in terms of human life and socioeconomic well-being, it is no surprise that attention on mRNA increased significantly following its emergency use authorization as a relatively rapid and effective vaccine technology. Now, as we look to the future of vaccine development, these characteristics of mRNA have become a priority in many vaccine research programs throughout the biopharmaceutical industry and will continue to be driven by major technological and research investments. These programs include research on mRNA technology for diseases such as HIV, cancer, and influenza.
Spotlight On mRNA
As we’ve seen first-hand in the development of mRNA-based COVID-19 vaccines, the technology has the potential to be developed and moved into clinical trials more rapidly than other vaccine platforms.1,2 With traditional protein or viral vector vaccines, proteins from a pathogen are used to stimulate the immune system to produce a protective response, such as antibodies, against the pathogen.1 Those antibodies are then available to fight off the pathogen if an individual is subsequently infected.1 The production of these vaccines can both be costly and time-consuming.1
mRNA vaccines, on the other hand, work by giving the body instructions to make a protein from a particular pathogen; this protein is produced by the cell and subsequently stimulates the body’s immune response.2 The immune response actively protects an individual against the pathogen to which one was vaccinated while generating a memory response to help to protect into the future.2 While most protein vaccines largely generate antibody responses, mRNA-based vaccines can also generate strong cellular responses that can add a layer of protection to the immune response.2 Additionally, the RNA is produced synthetically and encapsulated in lipid nanoparticles, essentially employing the person’s own cells as a bioreactor without need of an exogenous protein antigen, which can greatly minimize the time required to manufacture these vaccines.1
The Next Generation Of mRNA Vaccine Technology
Seqirus, actively working in influenza vaccine development and manufacturing, has been researching the next generation of mRNA technology – called self-amplifying messenger RNA (sa-mRNA) – as a viable influenza vaccine technology for a number of years.
sa-mRNA vaccines, which include genetically engineered replicons derived from self-replicating, single-stranded RNA viruses, have the potential to not only give the immune system instructions to make a protein but also provide instructions to amplify the mRNA.3 This means sa-mRNA can trigger production of much more protein and more potent cellular immune responses than an equivalent amount of mRNA.3
In a preclinical study, a single dose of sa-mRNA vaccine that was equal to that of a standard mRNA vaccine generated a robust protective response in a challenge model and separately showed higher levels of cellular responses than the mRNA vaccine.3 Additionally, the new sa-mRNA vaccines may help to avoid side effects associated with high RNA doses caused by innate sensing and detection of foreign RNA that human immune systems evolved to fight against if a lower dose can be used.3
Another important advantage of mRNA vaccines is versatility, allowing them to be generated quickly by changing the sequence coding for an antigen of interest.4,5 sa-mRNA vaccines can be efficiently produced using the same technology, which could enable vaccine manufacturers to potentially develop more effective vaccines with a smaller dose and lower rates of reactogenicity, underscoring its application for both seasonal and pandemic influenza.3
The Complexities Of Influenza
The development of seasonal influenza vaccines is particularly challenging given that the circulating strains of the influenza virus change from year to year, in contrast to other infectious diseases that stay constant for generations.6 Due to the changing, endemic nature of the influenza virus, reformulating influenza vaccines annually and revaccinating children and adults every year is necessary to help protect our communities from this serious, and sometimes deadly, infectious disease. When the vaccine is well-matched to the circulating influenza viruses, the U.S. Centers for Disease Control and Prevention (CDC) reports that vaccination reduces the risk of influenza illness by between 40% and 60% among the overall population.6
Influenza is also very different from other infectious diseases, most notably the COVID-19 virus. For example, one key difference is that COVID-19 vaccines target a single strain of SARS-CoV-2, whereas seasonal influenza vaccines target four different strains of the influenza virus.6,7 Each of these influenza viruses antigenically change over time, creating an even greater challenge for vaccine technologies.
sa-mRNA Potential For Influenza
Based on preclinical data, sa-mRNA vaccines show promise to prevent influenza more effectively and consistently than traditional vaccines.3 However, there is still much work to be done before we can predict its long-term viability. What we do know is that influenza viruses are highly variable due to frequent antigenic shifts and that a quickly adaptable vaccine platform such as RNA vaccines could offer a viable solution to addressing shifts with considerable time savings.
Additionally, in the likelihood of an influenza pandemic, an ideal response platform would be one that:
- is capable of delivering processes that are amenable to automation,
- contains generic processes to manufacture vaccines against any influenza strain reproducibly,
- has a small manufacturing footprint with disposable equipment, and
- utilizes raw materials that can be co-located in a single facility to enable rapid progression from gene sequence to vaccine product.8
Current data show that sa-mRNA vaccines may be capable of delivering all of these attributes and can be easily adapted to accommodate new influenza vaccine candidates in a cost-effective manner.8 Seqirus’ pre-clinical results on sa-mRNA seasonal and pandemic influenza vaccine candidates have demonstrated promising outcomes, and the company is targeting the commencement of clinical trials in the second half of 2022.
The U.S. government has even shown interest in the technology; The Biomedical Advanced Research and Development Authority (BARDA), a division of the Office of the Assistant Secretary for Preparedness and Response (ASPR) within the U.S. Department of Health and Human Services (HHS) has selected Seqirus to develop two influenza A(H2Nx) virus vaccine candidates for assessment in a Phase 1 clinical study. One of these candidates will utilize the sa-mRNA platform.9
The Future of sa-mRNA
Although Seqirus is currently focusing on pursuing sa-mRNA technology for seasonal and pandemic influenza, the inherent ability of mRNA-based vaccines to be developed in a lab, standardized, and quickly scaled into production has the potential for much wider applications.1,2
After years of scientific exploration, there is still much more research that needs to be done; however, early indications show that sa-mRNA could be useful for the prevention and treatment of a wide variety of infectious diseases, including influenza.3 While the biopharmaceutical industry should not regard sa-mRNA as a one-stop-shop for the future of infectious disease protection and prevention, the success of mRNA technology in COVID-19 vaccines shows promise for the next generation of mRNA technology.
About The Author:
Roberta Duncan is vice president and mRNA program lead at Seqirus. She is responsible for the cross-functional, whole-of-company execution of the Seqirus mRNA program. She joined Seqirus in 2017 as head of business operations, shared services, and clinical compliance. In 2019, Duncan was promoted to executive director of R&D portfolio and program management & business operations, shared services. She has more than 23 years of global experience in program management, clinical development, asset strategy, business operations, and team leadership. She earned her B.A. in biochemistry from New York University, and a dual MBA in international management from Purdue University and Tilburg University.
- Blakney, A. (2021). The next generation of RNA vaccines: self-amplifying RNA. Biochem (Lond). DOI: 10.1042/bio_2021_142.
- Pardi, N., Hogan, M.J., Porter, F.W., Weissman, D. (2018). mRNA vaccines - a new era in vaccinology. Nat Rev Drug Discov. DOI: 10.1038/nrd.2017.243.
- Vigel, B., Lambert, L., Kinnear, E., et al. (2018). Self-Amplifying RNA Vaccines Give Equivalent Protection against Influenza to mRNA Vaccines but at Much Lower Doses. American Society of Gene & Cell Therapy. 10.1016/j.ymthe.2017.11.017
- Rosa, S.S., Prazeres, D., Azevedo, A., Marques, M. (2021). mRNA vaccines manufacturing: Challenges and bottlenecks. Vaccine. DOI: 10.1016/j.vaccine.2021.03.038.
- Ballesteros-Briones, M.C., Silva-Pilipich, N., Herrador-Canete, G., Vanrell, L., Smerdou, C. (2020). A new generation of vaccines based on alphavirus self-amplifying RNA. Curr Opin Virol. DOI: 10.1016/j.coviro.2020.08.003.
- Centers for Disease Control and Prevention (CDC). (2021). Vaccine Effectiveness: How Well Do Flu Vaccines Work? Retrieved from: https://www.cdc.gov/flu/vaccines-work/vaccineeffect.htm. Accessed January 2022.
- CDC. (2022). Understanding mRNA COVID-19 Vaccines. Retrieved from: https://www.cdc.gov/coronavirus/2019-ncov/vaccines/different-vaccines/mrna.html. Accessed January 2022.
- Hekele, A., Bertholet, S., Archer, J., et al. (2013). Rapidly produced SAM((R)) vaccine against H7N9 influenza is immunogenic in mice. Emerg Microbes Infect. DOI: 10.1038/emi.2013.54.
- This project has been funded in whole or in part with Federal funds from the Office of the Assistant Secretary for Preparedness and Response, Biomedical Advanced Research and Development Authority, under contract numbers HHSO100201800004I and HHSO100200900101C.