Q&A – The Paradigm Shift To Continuous Flow Processes: A Holistic View
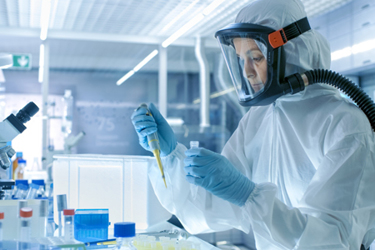
For pharmaceutical companies trying to optimize their manufacturing, finding a contract development and manufacturing organization (CDMO) capable of operating continuous flow processes at commercial scales is paramount to ensuring their eventual commercial success. To do so requires identifying partners with the expertise, assets, and skills to transition batch processing to continuous flow and scale up dangerous chemistries, as well as further optimize recycling and waste management.
Expertise in dangerous chemistries and continuous flow processes is crucial to ensuring the manufacture of safe, efficacious products while also delivering ecological benefits. In a recent webinar, “The Paradigm Shift to Continuous Flow Processes: A Holistic View,” Dr. Bert Metten, Technology Development Manager at Ajinomoto Bio-Pharma Services, explored Aji Bio-Pharma’s proven expertise and capabilities in handling dangerous chemistries.
At the conclusion of the webinar, Dr. Metten fielded questions on the intricacies of Aji Bio-Pharma’s approach to continuous flow, including its scale up parameters, modeling approaches, and other crucial decisions that impact reactor design and reaction optimization.
Q: Does Aji Bio-Pharma have experience with large molecule synthesis in flow?
Metten: Yes – we have experience, primarily within our other groups. In Japan, an example is protein refolding in flow. At the San Diego sites, we have experience with ADC conjugation in flow. Those are our large molecules we’ve made with the help of flow chemistry.
Q: Do you have experience in diazomethane reactions, other than diazomethane in cyclopropanations?
Metten: We mainly have experience in batch with diazomethane. For example, in esterification (methyl ester formation) as well as selective methylations of polyalcohol. With a special catalyst we could selectively methylate one alcohol with diazomethane, but most often the diazomethane is used in cyclopropanation steps.
Q: How was cleaning validation performed for this type of process? Is it similar to cleaning validation performed for campaign length?
Metten: For quality assurance, flow is not that different from batch. Just like in batch, you have an equipment train and area to clean. Therefore, you can use the same tools to follow-up what has been processed in the equipment and trace the cleaning, as well as the same tools to validate that the reactor is clean. Aji Bio-Pharma’s Cleaning Registration System is the ideal tool for that. The reactor that I showed in our case study, for example, is cleaned regularly, because we can observe some specific fouling over months in production, thanks to the in-line process analytical tools we have. We optimized the cleaning and have not observed problems since then.
Q: Could you talk about the application to functionalize polymeric nanoparticles?
Metten: Yes, our Japanese colleagues work with making nanoparticles utilizing natural products. The nanoparticles are made by a special mixture of large molecules in a buffer and the process requires very fast mixing. So micro-mixing in that case is really important to have a constant particle formation and to avoid aggregation of the nanoparticles. With these nanoparticles produced in flow, I think it’s also easy to have them functionalized in flow afterwards. Or, one can use pre-functionalized polymeric compounds to, for example, encapsulate special products or fragrance.
Q: Can you talk about the basis for choosing a 1-millimeter inner diameter (ID) to 4.5-millimeter ID?
Metten: The flow speed is dependent on the inner diameter size. In the lab, we chose to use a 1-millimeter inner diameter because we want to scale up. First, we want to provide our customer with kilograms and then scale further and where possible include the downstream process. We need several grams of material for this process development. The 1-millimeter diameter allows us to have the necessary productivity, and within a few hours, obtain the number of grams needed. We believe this is an excellent basis to further scale.
The 4.5-millimeter is more common in the first scale up to pilot scale. From there on, it really depends. We do safety calculations to determine how far we can go with the inner diameter. There are some equations we need to solve based on the kinetics, the heat exchange capability, and the onset temperature of the reaction. That last one dictates how far you can go with the inner diameter.
Further scale-up depends. If the 4.5 millimeter is the limit, then we have to do a numbering up from that. If we can go bigger, then we use a slightly bigger inner diameter as well. Therefore, it depends on what kind of flow rates we expect, and what kind of velocities. It’s important that we do not go too fast, but also that we don't go too slowly. We base ourselves on the reaction requirements as well.
Q: What are the scale up parameters that you consider when developing in flow from lab to pilot?
Metten: Safety studies play a huge role when determining how to scale up. It’s very important to have good familiarity with kinetics, especially when considering the heat being generated in the setup. We know what kind of inner diameter is needed and that’s how we identify what we are able to do. What can we use to scale up if the 1 millimeter is the limit or if the 4.5 millimeter is the limit? We don’t want to increase the inner diameter if possible, so it really depends. Sometimes it helps to use better equipment or materials, such as the silicon carbide reactor. There are some tradeoffs with the pressure drop. It’s mainly material, the speed of the reaction, and how far you can go with the diameter; those are really the most important parameters to consider.
Q: Do you take mechanistic or statistical models into account when designing reactors?
Metten: When designing reactors, we look at the kinetics, as well as perform statistical modeling to fine-tune the things that we want from the mechanistic modeling, so that we know how quickly everything will work and what the limits are of temperature overshoot. For example, if we observe a temperature overshoot of 10 degrees, and if that’s not acceptable, we redesign our reactor. We try to design our reactors according to what they will be used for. In other words, we don’t design our reaction to fit the reactor that we have – we do it the other way around.
Most people in flow processing will look at what the best, most optimal reactor is, and then try to implement that reactor into the pilot plant or in production scale. Many of the reactors on the market already help with that flexibility. Introducing fewer or more plates in-house, this allows us to design the lengths perfectly for our setup. As a rule of thumb, I always try to design a reactor in a pilot plant that could go up to one kilogram active per hour. Of course, in some cases it is incredibly diluted, and then it's not possible to get one kilogram an hour. But with the reactions I’ve done for clients and commercial productions in the past, that hasn’t been a problem. For example, for the reaction I showed in the case study, we created 50 kilograms in less than a day, which is 2.5 kilograms per hour active, and that was quite good – concentrated and relatively fast.
To learn more about The Paradigm Shift to Continuous Flow Processes: A Holistic View, watch the recorded webinar.