Picking The Best Virus Sample Prep Method In Process Development
By Friederike Eilts, Ph.D., chair of bioseparation engineering, Technical University of Munich
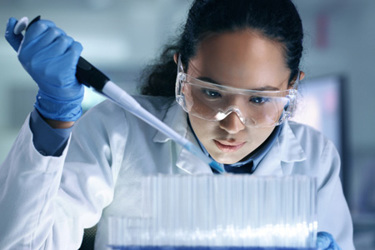
Often overlooked in viral vaccine development is that the development does not end with identifying an effective genotype; on the contrary, the production process, consisting of replication, purification, and formulation, needs adaptation for every new target. Throughout this process, a well-known bottleneck is an efficient purification, the downstream processing (DSP). Here, high losses due to low recoveries and virus inactivation often arise.
The first step toward adapting and optimizing a DSP operation is a thorough characterization of the target virus. This includes physicochemical characteristics like size, protein and lipid composition, hydrophobicity, surface charge distribution, and knowledge about stability and potency degradation. It should be noted that these characteristics may need reevaluation if the replication process, the upstream processing (USP), is adapted.1 Thus, characterization of the target virus is an iteration throughout the process development of USP and DSP, including formulation. This procedure relies on precise target characterization. To guarantee accurate virus characteristic results, most methods — especially of a physicochemical nature — rely on virus samples of high quality concerning:
- purity,
- concentration, and
- representability.
The purity corresponds to reducing host cell debris, protein, and DNA from production and product-related impurities, such as virus fragments, incomplete virus particles, or aggregates.
The concentration of the virus material indicates a suitable concentration range for subsequent studies. Viruses are produced in much lower concentration ranges than mAb production with titers in the g/L range. Thus, selectivity for concentration is essential.
Last, representability accounts for the similarities between the original virus stock of interest and the virus stock in the prepared sample. This also means that the selected method needs to prepare reproducible samples.
There’s No One-Size-Fits-All Preparation Method
The diversity of viruses in size and characteristics makes universal preparation methods hard to define. Thus, the preparation of samples for characterizing studies is intricate and needs careful evaluation for its suitability. Standard methods for lab-scale preparation are ultracentrifugation-based, often applying density gradients, e.g., sucrose or CsCl. Multiple time-consuming centrifugation steps must be undertaken to separate the virus particles from impurities. The advantage of this approach is the long history and broad knowledge of this method. Thus, the adaptability to new targets is simplified. Next to ultracentrifugation, precipitation and filtration are standard procedures. Although less specific, they stand out in their simplicity and easy adaptability. In the last few years, chromatographic methods have become increasingly important. Due to their high specificity, sample quality is very high. However, the adaptation is often laborious, and consumables are expensive. Combining chromatography with other methods like filtration as pretreatment can be a viable solution.
Several considerations concerning the preparation's aim should be made to choose the most suitable preparation method.
- How high does the purity of the sample need to be?
- How important is a high concentration of the target virus?
- Is separating different virus fractions, e.g., loaded from empty capsids, necessary?
Often, standard infectivity analysis needs no further preparation apart from sterile filtration or buffer exchange. On the contrary, preparations for physicochemical characterization of charge, hydrophobicity, and — depending on the method — size need to be of high purity, representing the target composition to a high degree. This is due to the high degree of interference with the less specific methods. For example, measurements to determine the virus charge are usually based on electrophoretic mobility, e.g., capillary electrophoresis, phase analysis light scattering, or tunable resistive pulse sensing. Impurities may interfere with the measurements by adding additional charges to the system or masking the virus surface. A striking example of the importance of sample purity for physicochemical characterization is a meta-study conducted by Michen and Graule on the isoelectric point (IEP), or point of zero charge, of different viruses.2 The variation between the measured IEP was up to several pH steps, depending on the purity of the preparations. Generally, the lower the sample purity, the higher the pH of the IEP. Such varying results can heavily impede decisions in the downstream process development depending on virus charge, e.g., ion exchange chromatography or precipitation. The preparation methods associated with low purity levels were often single-step, like precipitation. This is in accordance with another study by Shi and Tarabara3, who compared precipitation, centrifugal diafiltration, and CsCl density gradient centrifugation for their suitability to prepare bacteriophages. The centrifugation method prepared samples with the highest purity, resulting in the most accurate measurements of electrophoretic mobility and hydrophobicity. Precipitation and diafiltration, on the other hand, revealed contamination of co-precipitated proteins and aggregates, leading to deviations in IEP and hydrophobicity measurements.
Another study conducted in our group focused on comparing sample preparations by ultracentrifugation without using density gradients and by steric exclusion chromatography (SXC), which was, in some cases, followed by centrifugal diafiltration.4 The named methods were used to prepare the Orf virus vector, a dsDNA virus from the Poxviridae family that has been used for applications as a vaccine, oncolytic, and immunomodulatory agent.
The SXC is a relatively novel method that combines the advantages of conventional polymer precipitation, e.g., using polyethylene glycol (PEG), with chromatography. It is, like precipitation, based on crowding-out effects; however, the excluded viruses are retained at the stationary phase by the same crowding-out mechanism. Hence, there is no need for separating precipitates and bulk phase with a high chance of collecting co-precipitates. The adhered virus particles are eluted from the stationary phase only by omission of the PEG. By this, high degrees of contaminant removal are achieved, often reaching regulatory limitations within this single chromatographic step (below 100 µgprotein/mL). However, the concentration factors of infectious virus particles are by one to several orders of magnitude compared to ultracentrifugation.
In this study on Orf virus preparation, diafiltration increased aggregation of the virus and reduced the infectious titer. On the contrary, the samples prepared by SXC showed results comparable to those prepared by ultracentrifugation for size and electrophoretic mobility measurements, revealing monodispersity and virus particles of intact integrity. Additionally, a high virus infectivity recovery of up to 90% and the representation of the virus population was sufficient for successful degradation5 and formulation studies6 with the Orf virus.
Thus, the SXC overcomes the impurity challenge of classical precipitation while being a user-friendly and quick one-step method. To simplify the procedure, the SXC has been shown to work without a chromatographic system by using syringe filters and syringes.7 Previous studies on applying the SXC as a DSP unit operation included a wide range of viruses, e.g., adeno-associated virus (AAV)8, lentivirus9,10, hepatitis C11, influenza A virus12, baculovirus13, and koi herpes virus14.
SXC — A Purity-For-Concentration Trade-off
Exemplified by the variety of viruses the SXC has been applied to, the method broadens the range of choices for virus sample preparation intended for virus characterization. Although the method does not reach the high concentrations achieved using ultracentrifugation or precipitation, purities are exceptionally high. However, like precipitation, the adaptation of the procedure depends on multiple variables, such as target size and charge, the buffering system, the PEG composition, and the stationary phase.15 If no previous knowledge from SXC or precipitation experiments is available, estimates of the buffer composition based on the size and charge of the target virus need to be made. PEG is the most abundant substance in the system after water, so the focus should be on adapting this variable first. In most cases, to purify viruses with the SXC, the PEG concentration was chosen between 4% and 12%, using a molecular weight of 4,000 to 10,000 Da. Generally, the bigger the virus, the lower the PEG concentration and size needs to be. With this estimate, purity and infectious virus recovery might need pH, salt composition, and stationary phase optimization.
Conclusion
Preparing virus samples is a challenge that arises regularly throughout the development of viral pharmaceuticals. Choosing a suitable method relies on the sample’s purity, concentration, and representability needed for analysis. Another dimension of the choice is the simplicity and time consumption of the method. Next to conventional ultracentrifugation, precipitation, and filtration, chromatography can reduce the sample preparation to a single-step task. Here, steric exclusion chromatography combines the benefits of precipitation with chromatography, resulting in high-purity preparations suitable for physicochemical analysis and degradation and formulation studies.
Acknowledgments:
This article is based on the research undertaken at the Institute for Bioprocess Engineering and Pharmaceutical Technology, University of Applied Sciences Mittelhessen, published as articles Eilts et al. (2022), J Virol Methods 310, 114614 and Eilts et al. (2023), Vaccine 41 (32), 4731–4742. All co-authors of these articles are greatly acknowledged, especially Prof. Michael Wolff, Keven Lothert, Felix Pagallies, Ralf Amann, and Achim Rziha. The work was funded by the Heinrich Boell Foundation with a doctoral scholarship to Friederike Eilts. Additionally, an EXIST-Forschungstransfer grant (03EFKBW171) of the German Federal Ministry for Economic Affairs and Energy was granted, and the project was co-funded by the European Regional Development Fund as part of the Union’s response to the COVID-19 pandemic (IGJ-ERDF-Program Hesse - React EU 20008790).
References:
- Hämmerling F, Pieler MM, Hennig R, Serve A, Rapp E, Wolff MW et al. Influence of the Production System on the Surface Properties of Influenza A Virus Particles. Eng Life Sci 2017;17(10):1071–7. https://doi.org/10.1002/elsc.201700058.
- Michen B, Graule T. Isoelectric Points of Viruses. J Appl Microbiol 2010;18:290. https://doi.org/10.1111/j.1365-2672.2010.04663.x.
- Shi H, Tarabara VV. Charge, size distribution and hydrophobicity of viruses: Effect of propagation and purification methods. J Virol Methods 2018;256:123–32. https://doi.org/10.1016/j.jviromet.2018.02.008.
- Eilts F, Steger M, Pagallies F, Rziha H-J, Hardt M, Amann R et al. Comparison of sample preparation techniques for the physicochemical characterization of Orf virus particles. J Virol Methods 2022;310:114614. https://doi.org/10.1016/j.jviromet.2022.114614.
- Eilts F, Labisch JJ, Orbay S, Harsy YMJ, Steger M, Pagallies F et al. Stability studies for the identification of critical process parameters for a pharmaceutical production of the Orf virus. Vaccine 2023;41(32):4731–42. https://doi.org/10.1016/j.vaccine.2023.06.047.
- Eilts F, J Harsy YM, Lothert K, Pagallies F, Amann R, Wolff MW. An investigation of excipients for a stable Orf viral vector formulation. Virus Res 2023:199213. https://doi.org/10.1016/j.virusres.2023.199213.
- Marichal-Gallardo PA. Chromatographic purification of biological macromolecules by their capture on hydrophilic surfaces with the aid of non-ionic polymers. Universitäts- und Landesbibliothek Sachsen-Anhalt; 2019.
- Marichal-Gallardo P, Börner K, Pieler MM, Sonntag-Buck V, Obr M, Bejarano D et al. Single-use capture purification of adeno-associated viral gene transfer vectors by membrane-based steric exclusion chromatography. Hum Gene Ther 2021;32(17-18):959–74. https://doi.org/10.1089/hum.2019.284.
- Labisch JJ, Paul R, Wiese G, Pflanz K. Scaling Up of Steric Exclusion Membrane Chromatography for Lentiviral Vector Purification. Membranes 2023;13(2):149. https://doi.org/10.3390/membranes13020149.
- Labisch JJ, Kassar M, Bollmann F, Valentic A, Hubbuch J, Pflanz K. Steric exclusion chromatography of lentiviral vectors using hydrophilic cellulose membranes. J Chromatogr A 2022;1674:463148. https://doi.org/10.1016/j.chroma.2022.463148.
- Lothert K, Offersgaard AF, Pihl AF, Mathiesen CK, Jensen TB, Alzua GP et al. Development of a downstream process for the production of an inactivated whole hepatitis C virus vaccine. Sci Rep 2020;10(1):3018. https://doi.org/10.1038/s41598-020-72328-5.
- Marichal-Gallardo P, Pieler MM, Wolff MW, Reichl U. Steric Exclusion Chromatography for Purification of Cell Culture-Derived Influenza A Virus Using Regenerated Cellulose Membranes and Polyethylene Glycol. J Chromatogr A 2017;1483:110–9. https://doi.org/10.1016/j.chroma.2016.12.076.
- Lothert K, Sprick G, Beyer F, Lauria G, Czermak P, Wolff MW. Membrane-Based Steric Exclusion Chromatography for the Purification of a Recombinant Baculovirus and its Application for Cell Therapy. J Virol Methods 2020;275:113756. https://doi.org/10.1016/j.jviromet.2019.113756.
- Eilts F, Jordan LK, Harsy YMJ, Bergmann SM, Becker AM, Wolff MW. Purification and concentration of infectious koi herpesvirus using steric exclusion chromatography. J Fish Dis 2023(46):873–86. https://doi.org/10.1111/jfd.13800.
- Eilts F, Lothert K, Orbay S, Pagallies F, Amann R, Wolff MW. A Summary of Practical Considerations for the Application of the Steric Exclusion Chromatography for the Purification of the Orf Viral Vector. Membranes 2022;12(11):1070. https://doi.org/10.3390/membranes12111070.
About the Author:
Friederike Eilts achieved an M.Sc. Chemical and Biological Engineering in Braunschweig, working on adsorption processes in chromatography. Her Ph.D. focused on the downstream processing of viruses and viral vectors for pharmaceutical applications and virus characterization. With research stays in Waterloo, Ontario, and Vancouver, British Columbia, Canada; Bogotá, Colombia; and Troy, New York, she complemented her knowledge of viruses and chromatography. Currently, she is a post-doctoral researcher and the chair of bioseparation engineering at the Technical University of Munich. Friederike received several scholarships and awards of excellence throughout her studies and Ph.D., e.g., from the DAAD, the Heinrich Böll Foundation, and the VDI.