It's Time To Dethrone CHO When It Comes To mAb Production
By J. Casey Lippmeier, senior vice president of innovation, Conagen
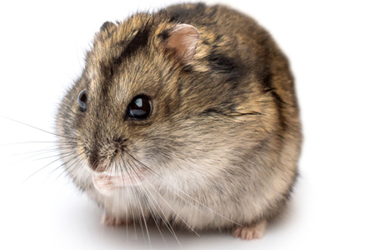
Monoclonal antibody (mAb) drugs and related derivatives have been a major boon for human health. Since the first one was commercially available in 1986 (muromonab-CD3), the FDA has gone on to approve more than 100 more. Today mAbs comprise nearly one-fifth of the FDA’s new approvals every year.1
The most common use of antibody drugs is for treatment of cancers or autoimmune disease, although their use for intervention against infectious disease is also common (with Regeneron’s REGN-COV2 antibody combination therapy being the most famous recent example). Keeping pace, manufacturing of mAbs has become tremendously advanced over the last four decades; however, most mAbs are still made using Chinese hamster ovary (CHO) cell lines. Several methods exist to coax these cells into producing mAbs, but the most preferred and productive techniques call for relatively expensive media compositions and large amounts of disposable materials.
For numerous reasons, production scales for CHO cultures are limited to volumes lower than 30 kL, which, consequently, limits the economies of scale possible from larger bioreactor volumes. The World Health Organization has cited the cost of producing CHO cell-produced mAb drugs as being in the range of $95,000 to $200,000 per kilogram.2 Compare this to the cost of producing human insulin and analogs from recombinant E. coli at $24,750 to $100,000 per kilogram or to food processing enzymes of lesser purity, like lactase from filamentous fungi, at just $40 to $50 per kilogram.3
Cost And Poor Scalability Are Blocking Patient Access
The high cost of production, limited ability to scale, and the entrenchment of CHO cell-specific manufacturing infrastructures for antibody-based drugs preclude broader access to these drugs. The total amount of monoclonal antibodies produced in the world today is just about 30 metric tons annually.4,5
If the cost of goods for mAbs could be reduced to $1,000 to $10,000 per kilogram, this annual volume could increase by ten- to one hundred-fold, and the superior safety and efficacy of this class of biopharmaceutical could be available to anyone who will benefit from them. However, radical changes will be required to amplify production volumes.
There are at least four distinct advantages that can be realized from lowered cost and broader access to mAb drugs. First and most obviously, greater uptake of these drugs by patients living in individual-payer systems becomes more feasible, as does their deployment in developing countries with single-payer systems that may not otherwise be able to afford them.
Second, drug rationing may be obviated. The best example of this came with the previously mentioned antibody therapy developed by Regeneron for treatment of active COVID-19 infections. During the height of the recent pandemic, this antibody therapy was reserved for only the sickest of patients, even though it would have been as useful an antiviral for anyone infected with COVID-19 as Tamiflu is for anyone suffering from influenza. The Regeneron therapy was only rationed because the company could not make enough of it quickly enough or at large enough scales to supply everyone who could benefit.
Third and fourth advantages include applications of antibodies beyond their use as human biopharmaceuticals. Antibodies, and particularly those of the IgA class, are important components of breast milk, which enables the best immune and overall physical development of newborn infants. These antibodies are, of course, not found in infant formula, and their bovine analogs in cow’s milk are denatured during pasteurization. Even if they were purified before pasteurization, the antibodies in cow’s milk are directed to bovine diseases, most of which will be entirely irrelevant to human health. Inclusion of individual or mixtures of mAbs in infant formula will require costs of production more in line with other food proteins.
And speaking of animals, mAbs for treatment of livestock and companion animals could be more readily deployed as well, as long as their prices can be lowered to the tolerance levels of those markets. The biggest players in veterinary drugs have already started on this path, with recent approvals being granted by U.S. agencies for a handful of antibody drugs where the cost/benefit proposition makes sense. Lower costs of production will broaden the proposition for many more of the 200 or more new veterinary drugs in R&D pipelines.6
But What About Glycosylation?
While lowering cost and increasing scales of production are both noble goals, one cannot achieve them with any sacrifices to safety or efficacy. For certain therapeutic effects, mAbs must carry an N-glycosylation, usually at the conserved amino acid Asn-297. This glycosylation enables proper binding to Fc receptors of effector cells, causing a cytotoxic effect to be exerted on the cancer or other target cell. The degree to which this glycosylation mediates serum half-life varies from one mAb drug to another, but this should also be considered during mAb design. Less specific O-linked glycosylations may have an overall impact on mAb structure and, thus, its function, but, more importantly, both kinds of glycans have a limited set of glycoform structures that are tolerated by human immune systems.
More economical alternative host systems for expression and production of mAbs either do not natively possess glycosylation machinery at all or will only glycosylate incompletely with glycoforms that may induce human immune responses. The simplest way to partly avoid this problem is to modify the DNA encoding the Asn-297 glycosylation site to another amino acid to prevent N-glycosylation entirely. For unmodified, “naked” antibodies, this may only be useful for a few cases where serum half-life is not adversely affected and Fc-receptor-mediated functions of effector cells are not needed. In fact, many FDA approved derivatives and fragments of antibodies are already made using microbial hosts for exactly this reason.
While naked antibodies remain important for many applications, the fastest growing class of antibody drugs are those that are formulated as antibody-drug conjugates (ADC), usually for cancer treatment. With this class of drug, the antibody is covalently attached to a cytotoxic agent, like a radioisotope or small molecule toxin. In this way, cells bearing a specific marker ligand recognized by the antibody can be destroyed while reducing or eliminating many of the safety issues and side effects of conventional untargeted chemotherapeutics. ADCs generally do not require an effector cell function to kill cancer cells, and therefore the N-glycan is dispensable. It may even be beneficial to use aglycosylated antibodies for ADCs as doing so may reduce the potential for off-site binding of the ADC to Fc-receptor bearing cells.
Let’s Start With A Common CHO Alternative — E. Coli
The most commonly used alternative host for making mAbs is E. coli, and the cost of production from this host is lower than it is from CHO cells. This bacterium does not natively possess a glycosylation system that will decorate mAbs, which, as mentioned, may be useful for certain applications. However, the cost reductions realized from the upstream portion of the manufacturing process (cultivation in a bioreactor) are offset by the higher costs of the downstream process (protein extraction, purification, and refolding).
E. coli usually sequesters proteins in inclusion bodies or the periplasm. The bacteria also produce a highly immunogenic endotoxin. Both challenges require significant downstream processing beyond what is required of mAbs secreted to the extracellular space by CHO cells, reducing the cost savings of the overall process.
Fungal Filaments And Yeasts
Filamentous fungi and yeasts are also alternative host systems that may be useful. Like all microbes, cultivation of fungal filaments or yeasts in bioreactors is also much more scalable than CHO cells (by a factor of about 10). In fact, the first ever mAb to be produced by a yeast for a pharmaceutical application recently won approval by the FDA and EMA. Eptinezumab-jjmr is an aglycosylated mAb produced from Komagataella pastoris (formerly Picha pastoris), used for treatment of migraines by infusion.7
Although this antibody bears no N-glycosylation by design, it will bear some O-glycosylations specific to fungi. Human IgG1, the most common class of antibody used for mAb therapeutics, does not carry any O-glycosylations.
In the case of eptinezumab the presumptive O-glycosylations attached by the yeast host did not present a problem to outweigh its benefit, but that may not be true for all future fungi-produced mAbs. Conversely, as compared to E. coli, fungi do not contain immunostimulatory endotoxin, and mAbs are secreted to the extracellular space, reducing the effort (and cost) needed to refold or purify unfolded mAb.
Although the cost of production of eptinezumab is not publicly available, it may be the lowest among all approved full-length mAb therapeutics.
Lesser-known Alternatives Under Development
Other systems under development for the manufacture of therapeutic mAbs include the whole zoo of microbial and multicellular hosts, as well as cell-free methods like direct RNA delivery. Each has its advantages and disadvantages.
Thraustochytrids
Thraustochytrids such as Schizochytrium and Aurantiochytrium are an emerging class of eukaryotic microbes that are unrelated to yeast but are cultured in a similar way. Most notably, they are reported to lack any O-glycosylation machinery, while also having simpler N-glycosylations that have been manipulated into human-compatible glycoforms.8,9
Thraustochytrids have been used for two decades to produce the nutritionally important omega-3 fat DHA, which is primarily used to fortify infant formulas and aquaculture feeds. Thus, these organisms already have well-established manufacturing technologies for economical fed-batch cultivation in bioreactors of more than 200,000 liters.10
Pseudomonas fluorescens
Among the bacteria, Pseudomonas fluorescens may be the most promising alternative to E. coli for expression of mAbs and mAb fragments or derivatives. In this microbe, high titer extracellular secretion of properly folded, soluble protein is much more easily enabled.11
Plant-based production
Other systems for mAb production should be noted here as well. First, certain crop plants like tobacco can be used to produce mAbs and related molecules in their leafy tissue. Higher plants are also capable of producing O-glycosylations and complex hybrid N-glycan structures, but in forms that may be immunogenic to humans. Here, too, however, N-glycans have been humanized via genetic manipulation. Unfortunately, due to the need for GMP-pharma manufacturing in greenhouses, the massive scalability of open-field cultivation is not feasible.12 Additionally, the downstream extraction of protein from the plant tissue and consequent purification processes will be much more intensive than it is from simpler cell culture supernatants, offsetting the lower cost of plant cultivation.
The human body
Another option leverages lipid nanoparticle technologies used for delivery of translatable RNA, which have recently seen tremendous advances due to their rapid deployment and use as vaccines for COVID-19. In this case, RNA encoding a naked antibody turns the patient’s body itself into the factory for mAb production. Although this technology may be limited to interventions that cannot be better addressed with an ADC, several clinical trials are currently underway to test various interventions for cancer and other diseases. Collectively, it is not yet clear if this approach will enable expression of effective or economical doses of translated mAbs, but it is very promising nonetheless and should be closely followed.
Microbial Systems Tend Toward Greater Efficiency In General
High titers of expressed mAbs may not be as critical for lowering cost using microbial systems as it is for CHO cell processes. Technoeconomic models constructed in my own lab suggest that continuous processes are the most cost-efficient for either CHO cell or microbial culture, but the productivities at which diminishing returns on cost reduction are realized are much lower with microbial culture than for CHO cell culture.
Depending on the downstream process used, overall production costs could be five- to tenfold lower. In general, microbial systems may be the most economical due to their scalability, potential to express high titers in low cost, often fully defined media, and lowered burden for downstream clearance of potential adventitious agents.
Parting Thoughts
There are, of course, other factors that lead to the high cost of biopharmaceuticals, which are beyond the scope of this article, not the least of which includes the requirements for approvals of biosimilar drugs in the U.S.
Innovator biopharmaceuticals and small molecule drugs rightly need to recoup the costs of their discovery and clinical research from product sales. But small molecule generics only need to prove that they are of sufficiently similar composition and structure for FDA approval. Biologic drugs like mAbs must additionally show equivalent clinical outcomes. Even if a manufacturer of a mAb biosimilar can demonstrate that all critical quality attributes fall within the lot-to-lot variations reported by the manufacturer of the innovator mAb, the FDA has prejudged this evidence as insufficient to ensure equivalent safety and efficacy on its own.13
To state it plainly, biosimilar manufacturers do not have to worry about recouping investments in discovery, but they still must recoup the cost of clinical development, which again leads us to consider other aspects of cost that can be reduced to affect pricing of mAb drugs. The trend is promising, however. Between 2015 and 2022, some 33 biosimilars were approved, 21 of which were available in the U.S. market.14
Monoclonal antibodies are useful drugs to which access should be expanded through reductions in price. Manufacturing technologies that are well-developed in other industries (mainly food and agriculture) are poised to help push these costs down. However, there must also be a business “pull” to justify the investments required in the GMP-pharma manufacturing facilities that need to be built to accommodate enabling alternate host systems. That in turn requires a certain boldness and risk tolerance from investors and disruptive innovators alike.
But, as mentioned, there are market opportunities in infant formula, veterinary health, infectious disease, and developing countries for lower-cost mAbs that are underserved or completely unserved at present.
Addressing these markets will lead to broader acceptance of the use of alternate host systems and their cognate manufacturing infrastructures over time and we are only now just taking the first steps.
Editor's Note: This article has been updated to explain that many factors limit CHO production volumes to 30 kL. A previous version said that shear forces limit production; however, shear may not become a meaningful limit until production volumes reach around 100 kL.
References:
- Mullard, A. (2021). FDA approves 100th monoclonal antibody product. Nature reviews. Drug discovery, 20(7), 491-495.
- Call for consultant on monoclonal antibodies for infectious diseases. (2021) https://www.who.int/news-room/articles-detail/call-for-consultant-on-monoclonal-antibodies-for-infectious-diseases
- Guerrand, A. (2018). Economics of food and feed enzymes. In Enzymes in human and animal nutrition: principles and perspectives. Academic Press.
- IAVI and the Wellcome Trust, Expanding access to monoclonal antibody-based products: a global call to action. (2020). https://www.iavi.org/features/expanding-access-to-monoclonal-antibody-based-products-a-global-call-to-action/
- Ecker DM, Seymour P. (2020). Supply and demand trends: mammalian biomanufacturing industry overview. Bioprocess Int. 18, 10–14.
- Jacob, J., (2023) Monoclonal antibodies show promise as new therapy for veterinary patients. J Am Vet Med Assoc 262(1).
- NDC Code(s): 67386-130-51, 67386-130-91 https://dailymed.nlm.nih.gov/dailymed/drugInfo.cfm?setid=79065861-6aa5-4d1f-829f-3a6471286b36
- Bayne, A. C. V., Boltz, D., Owen, C., Betz, Y., Maia, G., Azadi, P., ... & Lippmeier, J. C. (2013). Vaccination against influenza with recombinant hemagglutinin expressed by Schizochytrium sp. confers protective immunity. PLoS One, 8(4), e61790.
- Dahmen, J., Vermeulen, A., Payne, S., & Lippmeier, C. (2023). Thraustochytrid hosts for expression of proteins relevant to SARS-CoV-2 intervention. PloS One, 18(4), e0283592.
- Barclay, W., Weaver, C., Metz, J., & Hansen, J. (2010). Development of a docosahexaenoic acid production technology using Schizochytrium: historical perspective and update. In Single cell oils (pp. 75-96). AOCS Press.
- Squires, C. H., Retallack, D. M., Chew, L. C., Ramseier, T. M., Schneider, J. C., & Talbot, H. W. (2004). Heterologous protein production in P. fluorescens. BioProcess Int, 2(58), e58.
- Whaley, K. J., & Zeitlin, L. (2022). Emerging antibody-based products for infectious diseases: Planning for metric ton manufacturing. Human Vaccines & Immunotherapeutics, 18(2), 1930847.
- Makurvet, F. D. (2021). Biologics vs. small molecules: Drug costs and patient access. Medicine in Drug Discovery, 9, 100075.
- 2022 Biosimilars Report: The U.S. Journey and Path Ahead. https://www.cardinalhealth.com/content/dam/corp/web/documents/Report/cardinal-health-2022-biosimilars-report.pdf
About the Author:
Casey Lippmeier, Ph.D., currently serves as the senior vice president of innovation for Conagen Inc., a biomanufacturing company. He is an inventor of several technologies primarily focused on strains and processes for the production of nutritional products and biopharmaceuticals. He serves on several corporate advisory and journal editorial boards and currently lives in Southern California.