Incorporating Excipients Into QbD Studies For Drug Development — An Introduction
By Brian Carlin (DFE Pharma), Chris Moreton (FinnBrit Consulting), Dave Schoneker (Black Diamond Consulting), Katherine Ulman (KLU Consulting), Joseph Zeleznik (IMCD)
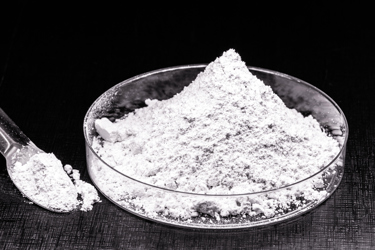
This article is the first in a three-part series based on information from the new guide Incorporation of Pharmaceutical Excipients into Product Development using Quality-by-Design, published by the International Pharmaceutical Excipients Council Federation (IPEC Federation).1
The International Conference on Harmonization’s (ICH) guidelines related to quality by design (QbD) — primarily ICH Q8, as well as Q9, Q10, Q11, and Q122-6— are high level and focus on the active pharmaceutical ingredient (API) and finished drug product. Applying API logic to excipients can lead to counterproductive adaptations inconsistent with QbD principles.
Silverstein7 contrasted the control of API quality versus that of excipients.
“API quality is improved by reducing the presence of all materials other than the desired chemical…. Extraneous substances may be harmful to the patient in that they may lead to side effects, or they are inert, thus reducing API purity and thereby compromising efficacy.
Excipient quality is described quite differently. While one would again refer to compliance with the compendial monograph (if there is one) or the manufacturer’s specification, a higher assay is not always better. While this may seem counterintuitive, excipients are often complex mixtures that include constituents arising from raw materials, catalyst, solvent, initiator residue, or side reactions. The International Pharmaceutical Excipients Council (IPEC) refers to these other unavoidable substances in the excipient as concomitant components. The performance of many excipients in the drug formulation may rely on the presence of such substances in the excipient. Concomitant components in the excipient may aid in solvating drug components, improving excipient functionality, etc. Excipient quality, therefore, is characterized as compliance to the monograph or specification and having a consistent concomitant composition.”
The pejorative illogicality of the excipient “impurity” is illustrated by non-crystallizing sorbitol syrup, which contains oligomers. Substitution with a purer crystallizing grade renders the finished product unusable if the patient cannot open the bottle due to cap locking. So-called excipient “impurities” are often essential to functionality or performance, hence the preferred term concomitant components.
APIs are synthesized for pharmacological effect, whereas most excipients have multiple applications beyond their pharmaceutical uses. For example, HPMC is a binder in wall board and cellulose is used in paper and viscose production. Pharmaceutical applications may be a very small portion of an excipient’s usage.
Traditional pharmaceutics focused on the functionality of the excipients, which drives the choice and level of incorporation of the excipients in formulation. The formula tended to be frozen early in development, once clinical and stability data accumulated. Scale-up often confounds small-scale data and the end result is poor manufacturability, where the finished product is not robust with respect to the inevitable variability in raw material.
Moreton8 defined a robust formulation as being “able to accommodate the typical variability seen in API, Excipients and Processes without compromising the manufacture, stability, performance or any other attribute of the product critical to the patient’s care or well-being."
In QbD, the emphasis shifts to the impact of excipient variability, which is a potential major (not exclusive) source of finished product performance problems. Excipient attributes can vary from batch to batch and from supplier to supplier. Not all attributes will be on the specification, so some variabilities may not be apparent.
Excipients are inherently variable, as they are not controlled at an individual parameter level. There are many degrees of freedom associated with polydispersity of particle size, molecular weight, and/or chemical composition, all of which may be process/supplier dependent. Particle size is usually controlled as an average or distribution, and dilute solution viscosities are often used as surrogates for molecular weight distributions. The composition profile of a complex mixture may not be well defined and the impact of the many components on performance is poorly understood. Excipient manufacturers can control excipient variability only within the limits of their process capability and for which the processing equipment was designed and constructed.9
There are many degrees of freedom associated with the excipients and the products into which they are formulated. The application of simple rules, such as compliance and fixed formulae, can give rise to unanticipated emergent behaviors, which is the definition of complexity.10 Emergent behaviors are another name for special cause variation.11
Factoring excipient variability into design of experiments (DoE), control strategy (CS), and life cycle management (LM) will increase finished product robustness.
Measuring The Impact Of Excipient Variability On Finished Product Quality
Once the prototype formulation and manufacturing process have been developed, emphasis shifts to assessing impact of excipient variability on finished product quality. Excipient suppliers and users (pharmaceutical manufacturers) should identify and, if necessary, control relevant excipient material attributes, some or all of which may not be included in pharmacopeial or excipient manufacturer’s specifications. Reliance on compliance with pharmacopeial or supplier specifications alone may not be sufficient for QbD, as compliance does not warrant fitness for purpose in a particular pharmaceutical product.
QbD seeks to minimize the risk that variability will adversely affect finished product quality. The impact of excipient variability will depend on the excipient’s role in the formulation and the finished product CQAs. Formulation and process development scientists should anticipate lot-to-lot and supplier-to-supplier variability in excipient properties and address the following potential areas of concern, which are novel concepts in terms of traditional formulation development:
- unknowns and uncertainty
- complexity
- common vs. special cause variation
- finished product criticalities
- excipient, process, and product drift
- ensuring representative sampling of excipient variability12
QbD places great emphasis on the management of risk. Once a risk is quantified, a decision can be made on tolerance versus mitigation of that risk using the criteria of severity, detectability, and probability. However, there may be unknown factors associated with both the raw materials and the finished product. Unknowns may be unknown to the user, the excipient manufacturer, or both. Risk management requires that the impact of unknowns (uncertainty) be minimized by involving all stakeholders, including excipient suppliers. Excipient unknowns include:
- composition and its impact on functionality and performance
- attributes not included in pharmacopeial specifications
- variability
- potential for interaction with latent criticalities in the finished product
Unknown does not mean unknowable. What may be unknown to users may be known to excipient makers and thus discoverable in discussion with the maker. Justification of reliance solely on pharmacopeial specification should include the results of such discussions.
Excipient complexity, and products into which excipients are formulated, should not be underestimated. A definition of complexity, which is very relevant to excipients in QbD is:
“the repeated application of simple rules in systems with many degrees of freedom that gives rise to emergent behavior not encoded in the rules themselves.”10
Pharmaceutical product development, control, and regulation have traditionally relied on simple rules, such as fixed formulae and fixed processes and (over-)reliance on pharmacopeial compliance. The many degrees of freedom (variability) associated with excipients are complemented by additional degrees of freedom from the processes and the finished products themselves. The resulting unpredictable emergent behaviors means that the excipients (and API), even if fully compliant, represent a reservoir of potential special cause variation. Finished product quality may unexpectedly and disproportionately become susceptible to the impact of a previously acceptable excipient variability within historical norms.
Common Cause Variation (CCV) Vs. Special Cause Variation (SCV)
CCV is the intrinsic noise or variation due to phenomena constantly active within the system. CCV is predictable probabilistically from a historical experience base and is characterized by irregular variation around a mean with no significance in individual high or low values. CCV data show zero trend (no time or batch dependency).
The term “special cause” was established by Deming,11 and such variation is characterized by:
- new, unanticipated, emergent behaviors
- inherently unpredictable
- outside historical experience
- potentially, inherent change in the system.
SCV is attributable to a specific disturbance. Removing all special causes leaves the intrinsic system noise, CCV. Deming’s reference to inherent system change is consistent with finished product criticalities. The type of variation is important to corrective and preventive action (CAPA).
Due to the complexity of excipients, and the products into which they are formulated, excipients represent a reservoir of special cause variation in the finished product, which must be addressed by the designer. As SCV is, by definition, unpredictable, it is not experimentally accessible during development and must be factored into the CS. Paradoxically, the more rigid or fixed the system is, the more susceptible it is to the impact of excipient variability. Flexibility built into the system to cope with SCV then becomes a criterion of design quality. There is little benefit in having products that work perfectly so long as nothing changes. Products are subject to cumulative changes throughout their life cycle. Serial univariate changes (even under change control) often lead to eventual failure, as quality is a combination of multivariate attributes. The failure need not correlate with a so-called “critical excipient.” It could also correlate with an attribute of a so-called “non-critical” excipient, within its norms of variability.
Finished Product Criticalities
A criticality, or critical transition, is defined as “being in a state, or at a point, where some quality, property, or phenomenon undergoes a definite change.”13 An example of criticality is critical micelle concentration, where the properties of dilute solutions below critical concentration are not predictive of the micellar system above, and vice versa. Another example is polymer solution gelation, which occurs above a critical concentration. The dilute solution viscosities commonly used to characterize polymer excipients are not predictive of higher application-specific concentrations.
Criticalities may exist within pharmaceutical products, unknown to the designer, and are therefore also known as latent conditions. Criticalities, as points of transition from one state to another, were not included in the ICH definition of criticality but can be critical if encountered during production. Excipients may disproportionately impact CQAs and manufacturability if their variability interacts with product criticality. A previously unknown, unremarkable excipient variability may unexpectedly start to govern the transition from one state to another. This may be what Deming meant by “inherent change in the system” when describing special cause variation. Criticalities in pharmaceutical products are most commonly associated with percolation effects or conflicting technological objectives.
Percolation Effects
Percolation thresholds are common in pharmaceutical systems, especially in powder mixes and tableting physics. An example is increasing the water content in a water-in-oil emulsion. The oil is the continuous phase in which the water is dispersed, but at a critical water concentration, the system may invert to give an oil-in-water emulsion, where the water is now the continuous phase. Powder mixes may exhibit similar behavior with particles of one component (A) dispersed in another (B). As A increases beyond a critical concentration, or percolation threshold, the mix becomes a dispersion of B in A. If the properties of A and B are different, there may be a marked discontinuity in the properties of the mix. In his review of the application of percolation theory to powder technology, Leuenberger14 warns that:
“formulations which contain a component with a critical concentration, i.e., close to the percolation threshold, may lead to non-robust conditions during scale-up and during subsequent large-scale production activities.”
An example is a disintegrant in a hydrophobic tablet matrix, at a level just sufficient to provide a contiguous network for water wicking. Even a slight variation in content uniformity could render parts of the tablet batch non-disintegrating.
Leuenberger also gave an example of a percolation threshold in tablet hardness vs. relative density. Below the criticality, the granules disintegrate; above it, they do not. Force transmission, and the resultant densities, within a compact are not homogeneous and may be dependent on factors such as the tablet geometry.
Extreme cases may be described mathematically by a binary 0-1 step function where the system goes from one state to another with little or no warning; hence the term “explosive percolation: from impossible to inevitable, without ever visiting the improbable.”15
Conflicting Technological Objectives
Conflicting technological objectives are another source of criticalities. Balancing compactability, dissolution, and lubrication in a simple tablet formulation with a high load of poorly compactible drug may yield a very narrow operating region. Trying to balance too many multiple competing objectives within a narrow operating region will result in much greater susceptibility to excipient variabilities and unknowns.
Over-granulation is another example of conflicting objectives. Continuing the granulation beyond a certain point may provide better granule and tablet properties but often at the expense of dissolution. The more precise this endpoint, the greater the impact of excipient variability. Criticalities may arise from transitions among pendular, funicular, and capillary granulation states, which may be dependent on excipient variability, particularly with absorbent excipients such as cellulosics and disintegrants.
Proximity to performance margins or failure points also increases the risk from the impact of excipient variability. Good examples can be found with design-critical rate-controlling polymers in modified release. The higher the level of gelling-matrix-former in a hydrophilic matrix tablet formulation, the lower the impact from variability in the excipient attributes. If faster drug release is required, it is generally advisable to maintain a high level of a polymer having lower viscosity (molecular weight) rather than reduce the original polymer to a level where the impact of excipient variability is greater. Similarly, maintaining a high loading of a more permeable rate-controlling controlled release film-coating is preferable to reducing the level of a low permeability coating to the point where it is more susceptible to the impact of both the coating precision and variability of the excipient attributes.
This article has been adapted from the CPhI 2021 New Year Report: Post Pandemic Trends & Excipient QbD.
References:
- IPEC Fédération asbl Rue Marie de Bourgogne 52 - 1000 Brussels – Belgium
T: +32 (0)2 213 74 40 - M: info@ipec-federation.org - ICH Harmonised Tripartite Guideline Pharmaceutical Development Q8(R2), August 2009. https://database.ich.org/sites/default/files/Q8%28R2%29%20Guideline.pdf
- ICH Harmonised Tripartite Guideline Quality Risk Management Q9, 9 November 2005. https://database.ich.org/sites/default/files/Q9%20Guideline.pdf
- ICH Harmonised Tripartite Guideline Pharmaceutical Quality System Q10, 4 June 2008. https://database.ich.org/sites/default/files/Q10%20Guideline.pdf
- ICH Harmonised Tripartite Guideline Development and Manufacture of Drug Substances (Chemical Entities and Biological Entities) Q11, 1 May 2012. https://database.ich.org/sites/default/files/Q11%20Guideline.pdf
- ICH Harmonised Tripartite Guideline Technical and Regulatory Considerations for Pharmaceutical Product Lifecycle Management Q12, 20 November 2019. https://database.ich.org/sites/default/files/Q12_Guideline_Step4_2019_1119.pdf
- Silverstein I. Pharmaceutical Technology Feb 2016 www.pharmtech.com/view/excipient-quality-and-selection
- Moreton RC Functionality and Performance of Excipients in a Quality-by-Design World, Part4: Obtaining Information on Excipient Variability for Formulation Design Space, American Pharmaceutical Review (2009), 12, (5), 28 – 33
- The International Pharmaceutical Excipient Council “Qualification of Excipients for Use in Pharmaceuticals”, 2020. (www.ipecamericas.org)
- Christensen K and Moloney NR, (2005) Complexity and Criticality, Imperial College Press, London, UK, p. vii.
- Dr. Edwards Deming and Profound Knowledge – Part 2; https://www.spcforexcel.com/knowledge/deming/profound-knowledge-part-2
- The IPEC-Americas Quality by Design (QbD) Sampling Guide, 2016. (www.ipecamericas.org)
- Merriam Webster (2016), http://www.pharmabiz.com/ArticleDetails.aspx?aid=99405&sid=21
- Leuenberger H. The application of percolation theory in powder technology. Adv Powder Technol. 1999; 10: 323-352.
- Gastner M, Yale-NUS