How Ring's Single-Capsid Protein Approach Optimizes Viral Delivery
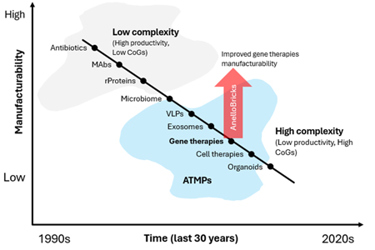
A conversation with Konstantin Konstantinov, Ph.D., Ring Therapeutics
Advanced therapies like gene and cell therapies have led to promising new treatments but have also created challenges in manufacturability. Traditional biologics like antibodies and recombinant enzymes are produced in highly optimized cell lines with well-established processes. That makes their production much simpler.
In contrast, new advanced therapy medicinal products (ATMPs) involve complex structures with millions of molecules, which cost more and are more difficult to produce at scale.
The solution lies in a return to biology, says Konstantin Konstantinov, Ph.D., chief technology officer at Ring Therapeutics. He's bullish on the critical role biology plays, in synergy with cutting-edge manufacturing technology, in enabling the industrialization of complex biotherapeutics. Ring's platform, based on a commensal anellovirus delivery system called AnelloVector, illustrates this refocusing on biology.
Konstantinov recently delivered a keynote presentation on creatively using biology to increase output and drive down the cost of goods during the International Symposium on Preparative and Industrial Chromatography and Allied Techniques (SPICA) conference in Milan.
Simplified product structures and high-yield cell lines are essential for efficient and cost-effective manufacturing processes, he says. This principle applies to various fields, including cell therapies, advanced vaccines, exosomes, and gene therapies.
Following his presentation, we had questions about his approach and more specifically about Ring's manufacturing platform, called AnelloBricks.
Let's start at the top. What do you mean by “biology must enable industrialization,” and what are the implications for ATMP development?
Over the last couple of years, we've observed an exponential increase in modalities that are not molecules anymore. We used to work with antibodies, with recombinant proteins, with enzymes, and so forth, which are produced in CHO or HEK cells. And the technology for this manufacturing is mature. But over the last 10 to 15 years, we've seen a rapidly growing number of modalities that are really complex, composite structures containing millions of different molecules.
They have incredible clinical value. They can do things that other modalities cannot do, and that's why there is such a dramatic interest in them. However, their increased complexity is resulting in more challenges for their manufacturing (Fig. 1). We often use the term manufacturability, and you can think of manufacturability as inversely proportional to complexity of the product.
While these modalities have great clinical value, we see a growing challenge in making them in significant amounts and at an acceptable price.
So, this is the challenge ahead of us. Now, how do we deal with it?
Fig. 1: The development of complex biotech modalities has resulted in reduced manufacturability, reflected by low productivity and high COG. The red arrow illustrates the improvement in gene therapy manufacturability with the AnelloBricks technology outlined in the text.
The synergy between biology and the manufacturing process is very important here because if you, for example, start with a cell line that is producing orders of magnitude less than what is needed, it doesn't matter what kind of incredible cutting-edge downstream or upstream technology you use, it will be tough to meet the requirements of high productivity and low cost of goods.
People like me — I'm a biochemical engineer responsible for process development and manufacturing— should do our job and push the process technology and the manufacturing technology all the time. However, to enable this technology to be efficient, we must have the right biology. This means well-designed product constructs. They should be as simple as possible, and they have to be highly potent.
In terms of cell lines, we also need cell lines that produce enough. Once you have the right biology, then the process development folks can do their job using cutting-edge process technology to achieve the goals in terms of productivity, cost of goods, and patient accessibility.
We tend to quantify productivity, what comes out of the process, in terms of mass — grams per liter, milligrams per liter, and so forth. When we transition to nanoparticles, the complex modality that I'm talking about, we transition to counting numbers of particles.
So, we transition from an analog way of quantifying our product to a digital way of quantifying our product. And it's an interesting way to see it: this is the transition from something that is relatively more simple or less complex to products that are significantly more complicated to make and to characterize.
How can the productivity lessons that monoclonal antibody development taught us help us to accelerate progress in ATMP manufacturing?
We may think, perhaps, about this in two parts. The first one is that monoclonal antibodies and recombinant protein manufacturing have evolved over the last 30 to 35 years in terms of production technology. We have developed processes, unit operations, and cutting-edge technologies that provide a really rich and powerful bioprocessing toolbox that can be used after proper adaptation for the manufacturing of some of these ATMPs. So this is one part.
The other part is that we learn a lot from monoclonal antibodies about the importance of biology. Over the last 25 to 30 years, the productivity of antibody in bioreactors was dramatically increased, likely by three orders of magnitude.
But if you think about how this actually can be broken down, maybe about 40% to 50% of this increase is due to process improvement, and a very significant portion of this improvement, maybe 50% to 60% qualitatively, is because of cell line improvement — you improve the biology.
That's not to say that only cell line improvement is going to address the productivity challenges for ATMPs, but it illustrates the significant importance of biology and how we need the combination, the synergy, of a powerful biology-enabling industrialization and cutting-edge manufacturing technology to achieve our goals.
You cannot go with one or the other. We need both.
Now having said that, I have to acknowledge that there is a challenge here and the challenge is that for all companies, the time to IND and BLA is critical. Companies are pushing for speed. Resources are limited, and we're expected to achieve a lot with less in terms of funding, in terms of time, and so forth. Therefore, we may not be able to develop early on 100% of the biology we need, but at least some core elements must be there to enable further improvements in a phase-appropriate manner.
Can you provide specific examples from your presentation of how industrialization-enabling biology has been successfully implemented in the development of complex biotherapeutics?
One example is the transition of manufacturing adeno-associated virus (AAV) gene therapies to stable cell lines referred to as "producers" where we don't have triple plasmid transfection, but the cell line provides stable inducible production of AAV vectors.
In recent posters and presentations, the Cytiva group has reported the improvement in productivity with such a cell line over 30x. That's significant.
Another example is what we did at my previous job at Codiak for large-scale manufacturing of exosomes with significant attention to the biology. What I mean is engineering the exosomes produced in HEK cells — engineering the HEK cells to make significant amounts of exosomes but also with the right exterior or interior payload decoration, resulting in high potency and 500-L process intensification.
With some of these examples, if you look at the manufacturing process from outside, the unit operations, the flow of the process, you can't tell whether we are making a complex modality like gene therapy or we are making antibodies, right? They look very similar.
But, of course, if you look closer at the unit operation, there are a lot of differences and advancements there.
What manufacturing advantages does the anellovirus have over more established viral vectors, such as AAV, and how does Ring exploit them with its platform?
I'm genuinely excited about this. I see unbelievable potential here.
I'll give you a high-level concept — why Ring’s approach is so different — then we'll talk about the implementation and address the issues of cost of goods and productivity in a relatively simple way.
With many of the new modalities, including gene therapy, we are asking the cells to produce the entire complex structure at once. Philosophically speaking, it is perhaps better to break a complex problem into pieces and solve the pieces one by one.
This is a high-level description of what we're doing with AnelloBricks.
Instead of doing the traditional triple plasmid transfection of HEK cells or mammalian cells and asking them to produce the complete gene therapy vector, we are actually using the biology of the anellovirus capsids to make things simple and modular.
Anellovirus has several distinctive advantages over known gene therapy vectors.
First, we know that it remains largely invisible to the immune system. It's because all humans have a lot of anelloviruses in our bodies, in our organs, and this is a commensal virus, so it sits without causing diseases.
This is fantastic because one of the issues with traditional AAV therapy is that it generates neutralizing antibodies. You give a shot once and then you cannot give another. Some people already have neutralizing antibodies that limit the applicability of AAV. Redosing is a major challenge.
Second is that we have different anellovirus serotypes, and so far, we have identified about 5,000 of them, residing in different organs. In every organ of the human body, the serotypes are slightly different. So, there is this natural tropism, preference for particular organs, and this gives us the opportunity to improve the delivery to the cells and the organs we want to target.
Lastly, there is one more advantage that may not look significant but is in fact very important. The capsid of anellovirus is simple. It is composed of a single protein. In AAV, we have three-capsid proteins, which are significantly more complicated.
With anellovirus, you have a single-capsid protein that encapsidates spontaneously in the presence of the nucleic acid. Instead of asking the cells to solve the entire problem and make the final product at once, we are breaking down the challenge into a couple of simple pieces. The first is to engineer the cell line to produce a single recombinant capsid protein like an antibody or enzyme.
Making a single recombinant protein is easy because we know the technology and you can produce significant amounts.
And then you purify this capsid protein, and then we combine this material with the desired payload, which at this point is a circular, single-stranded DNA. You combine the payload and the purified capsid protein under the right conditions, buffers, and so forth, and the capsid protein encapsidates around the payload forming the targeted AnelloVector.
And this happens spontaneously. So, you form the AnelloVectors containing the desired payload. You can see how the complex problem is broken down into several simple pieces.
This approach is also modular. You can imagine that we can use different payloads and just follow the same procedure. These payloads can be different DNA constructs or even RNA, because the capsid protein is looking for negatively charged nucleic acid — any nucleic acid.
So, how does this approach affect the cost of goods?
Here's an explanation of why the AnelloBricks platform is very efficient (Fig. 1). If you look at the stoichiometry, the conversion factor is impressive. One gram of capsid protein is equivalent stoichiometrically to e+17 capsids. That's a very large number.
To make a single recombinant protein, one gram is not difficult at all. Now, antibodies are made to 10 or 20 grams per liter, right? So, we don't intend to chase such large numbers, but in a bioreactor of a few hundred liters — I cannot imagine more than 500 L is going to be needed — you can produce a significant number of grams. When it's converted in this process into AnelloVector capsids, the quantities are just phenomenal.
Now, the conversion — one gram of capsid protein to e+17 — is ideal, 100% conversion. We don't expect 100%, and we're not going to get 100%. But we ran economic estimates based on this process to see what we can expect with an overall conversion factor of just 1%. We believe we can do much better than 1%, but based on this, we did a cost of goods comparison, and the numbers are just unbelievable. Let's just say that we are targeting a decrease in cost of goods by several orders of magnitude. So, yes, biology is a key enabler for industrialization!
About The Expert:
Konstantin Konstantinov, Ph.D., is chief technology officer at Ring Therapeutics. He has extensive experience in biomanufacturing, with a focus on process development and technology. He has led the development and commercialization of multiple products. His expertise in biomanufacturing is key to Ring Therapeutics' goal of advancing gene therapy.