Host Cell DNA Impurities: A Unique Challenge For rAAV
A conversation with Nic Preyat, UCB
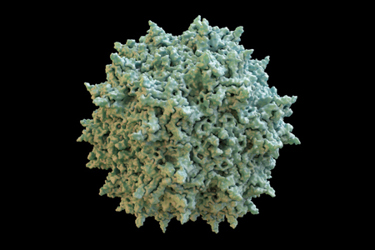
The production of recombinant adeno-associated viral vectors (rAAV) relies on host cells. This bioprocess is often associated with a high level of cytotoxicity, and the host cells are commonly lysed at the harvest step to maximize the recovery of the product. Hence, residual cellular DNA (rcDNA), or host cell DNA (hcDNA) is a process-related impurity that is released in large quantities during the production of rAAV. Nic Preyat and his colleagues at UCB have been studying hcDNA in rAAV gene therapy. This is an area of intense investigation for rAAV therapies compared to hcDNA in other biologics.
The potential safety impact of hcDNA impurities in rAAV gene therapies remains mostly unknown, and there’s a growing consensus in the community that established standards for viral vaccines and biologics don’t apply to rAAV products.
Preyat spoke about hcDNA encapsidation in November at the World Biomanufacturing Forum in Amsterdam. We had questions about his research and what’s at stake related to process development and patient safety.
Over the course of a few emails and a video call, Preyat helped us understand the challenges associated with hcDNA, how these can be mitigated, and why UCB is spending resources exploring this. His answers have been condensed and modified for clarity.
Can we start by talking about the risks of host cell DNA? Can you characterize them briefly? You note they're theoretical, with no known adverse clinical events. If that's the case, why are we worried about it?
During the manufacturing of viral vectors, including rAAV, significant levels of process and product impurities are generated. This includes heterogenous fragments of hcDNA, coming from the production cell system.
According to the literature and our experience at UCB, we can discriminate between four types of risks of hcDNA as an impurity:
- insertional mutagenesis resulting in the dysregulation of an oncogene or a tumor suppressor;
- cytosolic dsDNA-induced inflammatory responses via the cGAS-STING pathway;
- nuclear free DNA activation of p53-induced apoptosis; and
- immunotoxicity caused by neoantigens originating from random DNA insertion in a coding sequence.
These risks remain mostly theoretical, and more efforts will be needed to fully comprehend the actual risk level. A few years ago, in a briefing document on the toxicity risks of rAAV vectors for gene therapy, the FDA Cellular, Tissues, and Gene Therapies Advisory Committee concluded that it is difficult to set specifications for hcDNA since the risks are not clearly characterized. The regulatory landscape for hcDNA impurities is evolving. Historically, reference documents have set targets for hcDNA impurities in biologics and vaccines. These targets have been very much challenged and can be difficult to achieve in the context of treatments requiring high doses and/or highly concentrated drug products. For now, rAAV products with “high” hcDNA load have not shown association with clinical adverse events.
Moreover, differences in the analytical approaches to estimate hcDNA load could also result in differences in the reported hcDNA load between organizations. For hcDNA quantification, the current analytical methods are either quantitative PCR or digital PCR based on specific targets. These PCR methods are DNA sequence-specific and do not allow a quantification of all of the hcDNA impurities in the product. They rely on a correlation between the presence of these specific sequences and the total hcDNA impurities. The challenge is also that there is no universally accepted conversion factor available to convert copies of amplified DNA into mass units required by the authorities. As such, mass-based criteria are tough to conform to as they are inherently based on assumptions and correlations between copy and mass. In summary, the analytical approaches are not standardized, nor are relevant reference standards available to everyone in the industry. This results in challenges comparing hcDNA measurement results between products.
Differences in vector design and production systems may also lead to differences in product attributes that may play a role in triggering toxicities associated with AAV vectors. This is why it is believed that the specification for hcDNA should be product-specific.
Overall, product developers should have a risk-based approach that includes a solid characterization package to set specifications and ensure product safety.
We tend to think of impurity purification as a downstream function, but you are saying that the control of hcDNA impurities is part of an integrated control strategy in which both upstream and downstream functions have a role to play, correct?
Let’s start with the most obvious. On the downstream side, process development scientists have an arsenal of purification approaches that, when used appropriately, are efficient at clearing many process impurities. More specifically:
- Chromatography methods can help to clear efficient process impurities either leveraging specific product affinity or other physicochemical differences such as charge or hydrophobicity to separate.
- Tangential flow filtration is also extensively used to clean rAAV intermediate product prior and/or post-chromatography-based purification. Membrane pore size and operating conditions will influence the level of purification that you can achieve.
- Depth filters, typified by multiple porous layers, are generally more effective. Beyond the size of pores, these filters rely on adsorption, including the adsorption of hydrophobic residues, and sometimes charged molecules, such as DNA, are also selectively retained.
- Membrane filters are not always very efficient at removing hcDNA because they generally aim to filter particles greater than 0.2 micrometers — essentially, bacteria and large aggregates. But DNA, especially small DNA fragments generated during rAAV manufacturing, will go through the filter.
Then it is important to keep in mind that the total amount of process impurities generated during your upstream production will influence your downstream purification. It is therefore desirable to minimize the impurity level where possible. Not all impurities can be cleared during your purification, or only at the expense of your yields. This is why your process should be established as part of an integrated control strategy. While designing your process you should strive to limit the presence of hcDNA in the clarified harvest prior to the start of chromatography-based purification. Purely upstream process parameters can have a drastic effect on the total amount of hcDNA, or the size of the hcDNA fragments. The size of the fragments will potentially result in difference in ability to be purified, and cause toxicity. Typical upstream process parameters of importance to influence hcDNA impurity profile include the nature of the host cell line, the timing for the harvest, and the presence of endonuclease in the production system,
What are the limitations of process-based purification methods for removing encapsidated hcDNA from rAAVs? Why is it challenging to eliminate encapsidated hcDNA without affecting product yield?
My younger brother Ben is an unconditional fan of Sergio Leone's masterpiece, “The Good, The Bad and the Ugly,” which offers an analogy for rAAV and hcDNA impurities:
- The good is the desired rAAV product (capsid plus transgene) with a high purity.
- The bad is a mix of desired rAAV product with hcDNA outside of the capsid.
- The ugly are impurities in which hcDNA is encapsidated, which are very difficult to segregate from the desired capsid with a therapeutic transgene.
Typical filtration and chromatography methods are efficient at removing process impurities present outside of the viral vector capsid. It becomes more challenging to get rid of hcDNA when it is encapsidated. The packaged hcDNA is protected by the capsid against hydrolysis by process endonucleases (for example, benzonase). Moreover, with classical chromatography methods, it is complex to fully segregate capsids containing hcDNA from capsids containing a transgene, and you have to compromise between product purity and yields.
However, you ideally want both high yields and high purity. This invites you to explore technical avenues during your upstream production to minimize the proportion of vectors with encapsidated hcDNA. Your design space may be constrained by your cell line and your plasmid constructions in a transient transfection system.
Hence, among other approaches, you may want to focus on plasmid engineering and cell line engineering to further expand your design space and identify more appropriate conditions to limit hcDNA encapsidation.
Beyond load, what roles do hcDNA size and sequence play?
The field is moving fast and we are accumulating a lot of knowledge, but beyond the more generic quality attributes, scientists are still questioning which modality/product-specific quality attributes really matter.
In addition to hcDNA load, the size and the sequence of the hcDNA may also be relevant. It remains highly speculative, but one could make the assumption that encapsidation of a full-length oncogene could represent a risk of carcinogenesis in the unlikely event of recombination at a DNA strand break.
The attention for now is primarily on oncogenes derived from viral infection in human cells. Notably, adenovirus E1 in HEK293, SV40 large T-cell antigen in HEK293T, and E6/E7 human papillomavirus in HeLA cells are well characterized and should be monitored. For oncogenes of human origin, the good news is that rAAV can only package sequences of approximately 4 kb for double-stranded DNA.
Even if the coding sequence of some oncogenes falls within this limit, there are major differences between the length of genes and their corresponding coding sequence. For example, the main isoform of EGFR has a coding sequence of 3.6 kb, which corresponds to a gene length of 213 kb. The packaging of a human oncogene can consequently be considered as extremely unlikely.
Lastly, why are you and UCB focused on this issue right now?
At UCB Pharma, we are committed to continuously exploring the frontiers of science and technology to develop innovative solutions that best serve patients’ needs. We are committed to product safety and efficacy to improve lives of people living with neurological and autoimmune conditions. Our CMC development approach is very much guided by quality by design principles. We are using a systematic approach that aims to ensure the quality of gene therapy products by employing statistical, analytical and risk-management methodologies in the design, development, and manufacturing of our products.
A significant effort is therefore dedicated to the characterization of our rAAV to identify critical quality attributes (CQAs), including process-related impurities, and the critical process parameters influencing these CQAs. The Gene Therapy industry as a whole is still striving to refine the list of CQAs that most significantly influence the safety and efficacy of the products. These rAAV modalities are relatively new compared to antibodies or small molecules, so we have to face a series of open questions. We are influenced by “historical” guidance, and more recent guidance for advanced medicinal therapeutic products (ATMP).
Through connections in industry forums, we also realized that a large number of companies developing gene therapy products have levels of process impurities much higher than the thresholds defined in historical guidance. UCB is committed to developing high-quality products. In that context, it's important for us to invest time in characterization and process control studies to ensure quality and compliance of our products by design.
About The Expert:
Nic Preyat is an associate director of process sciences for the CMC development of gene therapy products at UCB Pharma in Belgium. Previously, he worked in various technical and leadership positions at Catalent Cell and Gene Therapy and, before that, at MaSTherCell. He received his Ph.D. in immunobiology from the University of Brussels.