Guidelines For mRNA Drug Product Manufacturing And Quality Control
By John F. Cipollo, United States Pharmacopeia
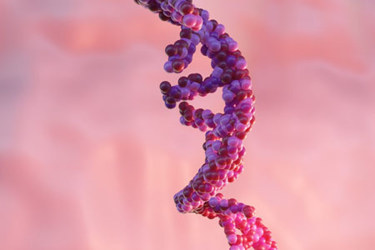
Messenger RNA (mRNA) is the template for protein synthesis. It serves as an intermediate to transfer the genetic information from DNA in the nucleus to the ribosome sites for translation to protein. It can encode virtually any protein, making it extremely attractive as a new modality for the development of vaccines, cell and gene therapies, oncology treatments, and other applications. The COVID-19 pandemic led to a massive collaborative effort to rapidly deliver vaccines against the SARS CoV2 virus. To meet this need, mRNA vaccines entered as a platform of choice due their inherent capacity for rapid development from design to drug product (DP). While mRNA vaccines have been under investigation for some time, none had been approved for use in humans prior to the pandemic. The entire industry interacted to expand production and delivery of vaccines, leading to an unprecedented pace of vaccine development and manufacturing.1
The success of the mRNA vaccines during the pandemic has increased interest in this platform. As the industry moves back to more predictable levels of production and demand, applications of mRNA for vaccines and therapeutics are projected to expand. Coupled with its ability to provide more rapid product delivery compared to other vaccine platforms, this modality has become highly attractive in drug development. As of February 2023, more than 140 clinical trials were using mRNA vaccines, with 70% focusing on infectious diseases, 12% in oncology, and the remainder targeting other gene and cell therapies.2 The platform is also amenable to continuous and advanced manufacturing, providing the potential for reduced cost.3 While the range of future mRNA products will differ in their targets, their manufacturing processes are highly similar, making them amenable to platform manufacturing, as recently described by the European Medicines Agency.4
As such, there is a need to build consensus on product quality attributes (PQAs) and test methods. Guidelines on analytical testing can therefore benefit not only COVID-19 vaccines but other mRNA vaccines as well as other mRNA-based drug products and ultimately form the basis of reliable documentary and physical standards to support the growing mRNA field.
The Growing Body Of Analytical Standards To Support mRNA Processing
To facilitate an industry-wide discussion and build consensus on product quality attributes and relevant test methods, USP engaged with manufacturers, national regulatory authorities, and other stakeholders. USP released the first edition of its draft guidelines, Analytical Procedures for mRNA Vaccine Quality, in February 2022. Over 300 public comments were received from a variety of stakeholders, including mRNA manufacturers, equipment vendors, raw material suppliers, and national regulatory bodies. Some common themes centered on requests to include analysis of starting materials, especially DNA template, and methods for analysis of DP and lipid nanoparticles (LNP). Since then, and through further interaction with experts in the field, USP released the second edition of the mRNA draft guidelines in April 2023, and reported advances therein at the 2023 World Vaccine Congress.
The updated guidelines include over 15 additional methods, including several that were donated to USP as part of the public comment process. The guidelines were expanded to include analytics for evaluation of DP with methods targeting the qualities of:
- identity,
- content,
- integrity,
- purity,
- potency, and
- safety.
Methods were introduced to analyze quality attributes of both the lipid components and the mRNA. These methods are listed in Table 2. Added methods for drug substance (DS) analysis included mass spectrometry-based methods to monitor 5’ capping efficiency and poly(A) tail length and heterogeneity. Other methods were added for monitoring impurities, including dsRNA, residual protein, and residual nucleotide triphosphates (NTPs). Drug product (DP) methods were also added to monitor LNP attributes including size, polydispersity, and encapsulation as well as extraction methods and other preparatory methods to render separation of lipids and mRNA components. Methods to evaluate RNA attributes including size, integrity, aggregation, and quantitation at the DP level were also added. Methods were also added for detection of expressed mRNA encoded antigen. Based on stakeholders’ comments, an overview of quality considerations for upstream plasmid production was also included.
This article provides perspective and insight on product quality attributes for mRNA vaccines and other mRNA-based products as they move into the future as viable pharmaceutical entities for preventive and therapeutic applications. In the following two sections, some of the history and properties of these drugs will be discussed, including their relationship to quality attributes, why they should be monitored, and the testing procedures in the guidelines that target these components as quality attributes.
mRNA Drug Substance Structure And Relation To CQA
The production of mRNA is performed utilizing an in vitro chemoenzymatic process. The mRNA has essentially four defined components, including 5’ cap, upstream and downstream untranslated regions (UTR), a coding region, and a poly(A) tail.
Initially, in vitro synthesis faced a major hurdle for incorporation of the 5’ cap. Natural Cap, commonly referred to as Cap 0, is not efficiently incorporated and can be oriented in correct or reverse orientation, making in vitro synthesis with Cap 0 unfeasible. Moreover, any modification of the cap or related processing steps to correct this issue must yield a cap that is not recognized as foreign mRNA, as these would be quickly destroyed by host defenses such as interferons, pathogen associated molecular patterns, pathogen recognition receptors, and other innate immune system responders.5 Therefore, the 5’ cap must closely resemble that of the host while overcoming synthetic issues. Alternative processes have been developed that overcome these barriers, such as methods that utilize anti-reverse cap analog (ARCA) and Cap 1. These avoid host defense issues while overcoming synthetic ones. Both incorporate efficiently in the correct orientation, although the former requires further enzymatic modification by 2’-O methyltransferase subsequent to mRNA synthesis. Cap incorporation efficiency must be monitored, and this can be accomplished using RP-HPLC and IP RP-HPLC methods described in the guidelines. Both of these methods can provide percent cap incorporation and can also be coupled with mass spectrometry to provide accurate mass and fine structural detail.
UTR length and structure serve as regulatory elements supporting translational efficiency. Optimization of the sequence in these regions can be performed with the use of UTR libraries. The Kozak sequence, derived from human alpha-globin RNA, used by BioNTech, is an example of one 5’ UTR used for translational optimization.6 A range of both 5’ and 3’ UTRs used in current mRNA vaccines have been reviewed.7 The quality of these regions should be monitored as they are critical to drug performance. The mRNA, including the UTR regions, can be monitored through methods that determine size and sequence of the mRNA. Methods are listed in Table 1.
The poly(A) tail length can affect the half-life and translation efficiency of the mRNA. Optimization of the length of the final poly(A) tail is a major focus during development. This moiety aids in transport within the cell and protects mRNA from nucleases in the cellular cytoplasm. Current mRNA vaccine production processes utilize a linearized plasmid as a template for mRNA. The size of the poly(A) tail produced can be limited by the total base pair capacity of the encoding plasmid. The closer to capacity the encoded mRNA, the less room available for the encoded poly(A) tail.
Other methods for polyadenylation can be used to add the poly(A) tail post mRNA synthesis, such as addition via PCR or by enzymatic synthesis by a poly(A) polymerase. Both the plasmid encoded synthesis and PCR addition methods result in a more uniform poly(A) tail, while poly(A) polymerase results in more heterogeneity.8 Regardless of the method used to generate the poly(A) tail, this attribute should be monitored as its length and heterogeneity will impact drug performance. These attributes can be monitored by a range of methods, such as IP RP-HPLC, as described in the guidelines. The method can also be hyphenated to mass spectrometry to provide more compositional and structural detail. A common method uses a non-specific ribonuclease such as RNAse H. A guide primer is used to target the enzyme to a specific region upstream to the tail. RNAse H will only cleave double stranded RNA. Thus, short oligonucleotides are produced from the upstream primer guided site yielding the region containing the tail, which can be quantitated to reveal percent of poly(A) mRNA and heterogeneity.
The mRNA size and composition are critical attributes that can be codon optimized for improved expression. The mRNA can be produced with incorporation of non-natural nucleotides such as 5-methyluridine and N6-methyladenosine to reduce degradation and immunogenicity and increase stability. The sequence and size can be monitored using methods designed to track these attributes, such as Sanger sequencing and high throughput forms of sequencing for the former and capillary gel (CGE) and capillary electrophoresis (CE) for the latter attribute. LC/MS methods can also be developed for intact mass analysis, which is useful for tracking modified nucleotide incorporation.
Table 1. Characterization and release testing for mRNA drug substance
mRNA Drug Product Characteristics And Relation To CQA
Based on traditional concepts of drugability, mRNAs are not favorable for use as pharmaceutical drugs as stand-alone entities since they are relatively high in molecular weight, highly charged, unstable under physiological conditions, and can produce unwanted immunogenicity. To overcome these properties, strategies have been created utilizing delivery systems that render these natural barriers inconsequential. Over the past 40 years, LNPs are one delivery method that has evolved to serve this purpose.
Lipids used in LNPs came through several decades of research. Cumulatively, over a thousand synthetic lipids have been investigated. Those with favorable pharmacological characteristics and safety profiles have advanced in drug development and production to deliver a range of pharmaceutical cargo, including mRNA.9 The size range is critical and will impact the pharmacokinetic qualities such as cellular uptake and flow through the lymphatic and other distribution systems.10
Currently, LNPs used in mRNA products have four components:
- an ionizable cationic lipid, which promotes self-assembly into virus-sized particles and facilitates endosomal release of mRNA to the cytoplasm,
- lipid-linked polyethylene glycol (PEG), which has been shown to potentiate longer half-life,
- cholesterol, a membrane stabilizer, and
- naturally occurring phospholipids or their close analogs, which support lipid bilayer structure.
Proper ratios and structural orientation are key to LNP function and are critical quality attributes. The lipid ratios and compositions can differ depending on the drug target, delivery method, and indication. These can be controlled through monitoring throughout the DP production process. Content and composition can be monitored by reversed-phase high-performance liquid chromatography (RP-HPLC) using charged aerosol detection (CAD), evaporative light scattering detection (ELSD), or diode array detection (DAD).
Typically, the LNP manufacturing process includes four steps: formation, size reduction, purification, and sterile filtration. The initial crude liposomal suspension and its size reduction can be achieved by either a solvent dilution followed by an extrusion process or by microfluidic processes. Extrusion is a pressure filtration procedure, where the lipid suspension is forced through a filtration process with a defined pore size. The overall process is designed to limit physical stresses such as shear forces and result in a uniform LNP composition and size. The size, polydispersity, and composition can affect LNP uptake and distribution and are therefore important quality attributes related to drug performance.11 The LNP/mRNA encapsulation process will render some portion of the mRNA outside the LNP lipid micelle. Unencapsulated mRNA will rapidly degrade and therefore does not contribute to vaccine efficacy. Size, polydispersity, and encapsulation can be monitored using a range of methods, examples of which are described in the guidelines.
The goal of the LNP system is safe and efficacious delivery of the encapsulated active cargo. To achieve these ends, the manufacturing process must deliver the entrapped mRNA while retaining critical qualities including the correct sequence (identity) at the appropriate concentration (content) while retaining integrity, purity, potency, and safety. Tests that could be employed for characterization and/or release are included in the second version of Analytical Procedures for mRNA Vaccine Quality and are shown below in Table 2.
Table 2. Characterization and release testing for mRNA drug product
Future Perspectives On mRNA Drug Standards
In response to the pandemic and to the emergence of mRNA as a new vaccine modality, USP worked with expert volunteers and other stakeholders to make available guidelines, methods, and educational materials to help build consensus and provide a framework to support mRNA vaccine quality. USP will continue efforts to deliver documentary and physical standards and tools in support of mRNA and other vaccine platforms in partnership with stakeholders and experts across the industry. Throughout the past several years it has become clear that mRNA manufacturing platforms will remain part of the growing pharmaceutical industry landscape, including infectious disease vaccines as well as cancer treatments and gene and cell therapies.
As of the fourth quarter of 2022, there were more than 20 mRNA-based drugs with either FDA approval or in late Phase 3 clinical trials. The mRNA therapeutics market is projected to expand considerably through 2030.12 The successful rollout of mRNA vaccines seen during the pandemic has fueled growth of products using RNA manufacturing platforms extending into a range of therapeutics applications. By way of example, 2021 saw the second highest number of mRNA vaccine trials targeting cancer. In 2023, industry sponsored mRNA vaccines trials increased by more than one-third compared to those in 2021.13 Clearly, mRNA drugs, including vaccines, are a growth area in the pharmaceuticals market.
References
- Kim, Y.C., B. Dema, and A. Reyes-Sandoval, COVID-19 vaccines: breaking record times to first-in-human trials. NPJ Vaccines, 2020. 5(1): p. 34.
- Newton, W., mRNA vaccines: Four major clinical trial readouts to watch in 2023. Clinical Trials Arena
- Alina Hengelbrock, A.S., Heribert Helgers, Florian Lukas Vetter and Jochen Stube, Scalable mRNA Machine for Regulatory Approval of Variable Scale between 1000 Clinical Doses to 10 Million Manufacturing Scale Doses. Processes 11(745).
- Agency, E.M., Guideline on data requirements for vaccine platform technology master files (vPTMF), C.f.V.M.P. (CVMP), Editor. 2022.
- Shanmugasundaram, M., A. Senthilvelan, and A.R. Kore, Recent Advances in Modified Cap Analogs: Synthesis, Biochemical Properties, and mRNA Based Vaccines. Chem Rec, 2022. 22(8): p. e202200005.
- Asrani, K.H., et al., Optimization of mRNA untranslated regions for improved expression of therapeutic mRNA. RNA Biol, 2018. 15(6): p. 756-762.
- Fang, E., et al., Advances in COVID-19 mRNA vaccine development. Signal Transduct Target Ther, 2022. 7(1): p. 94.
- Grier, A.E., et al., pEVL: A Linear Plasmid for Generating mRNA IVT Templates With Extended Encoded Poly(A) Sequences. Mol Ther Nucleic Acids, 2016. 5(4): p. e306.
- Naidu, G.S., et al., A Combinatorial Library of Lipid Nanoparticles for Cell Type-Specific mRNA Delivery. Adv Sci (Weinh), 2023: p. e2301929.
- Moon, J.J., et al., Enhancing humoral responses to a malaria antigen with nanoparticle vaccines that expand Tfh cells and promote germinal center induction. Proc Natl Acad Sci U S A, 2012. 109(4): p. 1080-5.
- del Pozo-Rodriguez, A., et al., Lipid nanoparticles as vehicles for macromolecules: nucleic acids and peptides. Recent Pat Drug Deliv Formul, 2011. 5(3): p. 214-26.
- mRNA Therapeutics Market Size, Share & Trends Analysis Report By Application (Infectious Diseases, Oncology), By Type (Prophylactic Vaccines, Therapeutic Drugs), By End-use, By Region, And Segment Forecasts, 2022 - 2030. 2022; Available from: https://www.grandviewresearch.com/industry-analysis/mrna-therapeutics-market-report.
- Newton, W. mRNA vaccines: Four major clinical trial readouts to watch in 2023. 2023; Available from: https://www.clinicaltrialsarena.com/features/mrna-vaccine-trials-to-watch).
About the Author:
John Cipollo, Ph.D., is a senior principal scientist and team lead at the U.S. Pharmacopeia. Previously, he founded and led the CBER Vaccine Structure Group, where the team focused on vaccine antigen structure and pathogen interaction with the host immune system. The group worked on mass spectrometry development and other analytical methods and informatics. Concurrent with his research, he served as a CMC vaccine reviewer for over 15 years. In his role at USP, Cipollo leverages his experience in vaccine research and review to help advance documentary and physical standards to support vaccine development and manufacture. His efforts include educational outreach to improve the industry’s understanding of the principles of vaccine development, production, and quality and their importance in human health.