4 Focus Areas To Improve AAV Production Lines
By Antony Hitchcock, principal and owner, AGH Bioconsulting
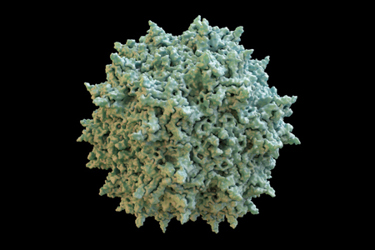
In my previous article, I took a holistic view of how it may be possible to address the challenge of treating large patient groups through a combination of improvements in vector design and manufacturing processes. In this article, I look more closely at manufacturing process development in pursuit of population-scale production.
There are arguably four key areas of focus around manufacturing processes.
- The first is the improvement of process robustness, as batch failures are a significant issue for suppliers and affect cost, materials supply, and regulatory compliance.
- Second, there is a need to increase productivity at cellular and volumetric levels and to maximize production scales.
- Third is the need to improve product quality in terms of batch-to-batch consistency, product purity, and product potency.
- Fourth, we have the cost of goods, which, to some extent is linked to reducing batch failures and increasing productivity but also production scales and approaches.
The development of biological production processes can be split into two key areas:
- the biology relating to the production of the product within the cell
- the physical production process itself
Obviously, developing an in-depth understanding of the production process biology, including the design of the production and the understanding and control of the cellular processes involved, is critical to producing the desired product and maximizing productivity. This is very much the case for viral vectors, where the goal is to maximize the production of intact, functional vectors, as opposed to non-functional, empty capsids, which present major processing and clinical challenges.
As adeno-associated viral (AAV) vectors are delivery vehicles for therapeutic genes, their production is, to a large degree, independent of the gene sequence they carry. This allows for production processes to be disconnected from one specific product. “Platformed” processes can be applied to multiple products. Consequently, much of the development work often focuses on optimization procedures, including the use of scale-down tools to allow new products to be produced within established production platforms and the process itself, rather than optimizing the production of a singular product.
When developing the biological elements of a process, there is a need for deeper knowledge of the biology around vector production and assembly processes. The development of this knowledge requires collaborations with academic groups and, while industry is getting better at forming these relationships, they may take several years to affect manufacturing processes. They also bring inevitable risks. Interestingly, we have seen the emergence of academic spinouts supporting these kinds of activities, which are likely to be more effective.
On the other hand, process improvements are largely dependent on in-house knowledge and the application of existing tools and developments. They often require development partnerships between producers and suppliers.
When it comes to implementation, some developments can be put into practice rapidly using existing technologies. Others require greater levels of development and investment, including development of the fundamental science and of new technologies and tools, which inevitably take significant time and money. The costs associated with process changes come from the extra labor, consumables, and lost facility revenues, and any potential gains must outweigh those expenses.
Figure 1 shows the areas ripe for process development.
Plasmid And Vector Engineering
Vector production within a cell line requires plasmid coding for the gene of interest, a helper function, the capsid proteins, and the vector replication functions. While there are significant efforts to improve vector function, it is also now recognized that there is room for improvement in the replication elements, giving the potential for improvements in productivity and product quality.
A second area for potential development is the modification of vectors to increase levels of secretion into the culture media. Many vectors are accumulated intracellularly, but we know that with certain serotypes there is a high level of secretion into the culture media and, more, recently the capsid sequences that enable secretion have been identified. It has been possible to modify different serotypes to significantly increase secretion levels.
This could have significant implications for product purity. In current processes, there is a need to release vectors using detergent. This is then followed by enzymatic digestion of nucleic acid ahead of clarification operations, which in turn reduces the levels of cellular contaminants being carried into downstream processes. Additionally, eliminating the cell lysis step simplifies the harvest process, reducing cost and improving product purity.
Cell Lines
Early vectors were produced in off-the-shelf, non-clonal cell lines acquired from cell line depositaries. More recently, manufacturers, including CDMOs, have established clonal cell lines for their production platforms. This potentially offers improvements in process consistency and titers. We also see both CDMOs and technology providers offering producer and stable cell lines as part of their service offering. While technically challenging and potentially costly, they do offer significant advantages with regard to eliminating the need for plasmid DNA to support vector production, bringing about significant cost savings and supply chain simplification. Plasmid DNA adds significant costs to vector production.
Cell Culture
The focus of development in this area is to manipulate and exchange media pre- and post-transfection to increase cell densities, improve transfection efficiencies, and maximize vector production post-transfection, which is increasingly performed with perfusion-based systems. As we learn more about the biology of vector production at a cellular level, both in transient and stable cell lines, this area is going to become an increasingly important approach to process improvement, including the use of metabolomics, as we have seen in antibody production processes.
Transfection
Transfection optimization is critical to successful vector production. It has been a key challenge for vector producers with regard to maximizing productivity, achieving process consistency, and scaling up.
In recent years, we have seen significant improvements in this area with regard to optimizing plasmid ratios and the development of new transfection reagents. We have also seen groups assessing key issues around the formation of plasmid complexes in terms of optimal sizes for cellular uptake and the requirement to control this. Key to success will be the identification of critical process parameters around this operation, routes for online monitoring, and control of complex formation in addition to culture systems.
Plasmid Harvest And Recovery
Most vector producers have established procedures for cell lysis and DNA digestion, with the key issue being the move away from Triton X-100 to more environmentally acceptable detergents. Filtration processes have seen some interesting developments such as the tangential flow depth filtration procedures, which allow for debris removal as well as volume reduction and buffer exchange. Improving the interface between recovery operations and the affinity capture step will be important to further scaling processes, especially when cell densities are increased. Greater cell densities potentially burden downstream processes in terms of host protein and DNA removal.
Affinity Capture
Affinity chromatography has now become the procedure to capture vector from the culture lysate. It is the main route to removing host proteins and DNA. The two potential areas for development are, first, the ligands themselves in terms of specificity, especially with regard to novel engineered vectors and robustness of cleaning and recycling of resins. The second is the format. Currently, the affinity ligands are presented on conventional porous resin beads, which are not ideal for purifying large structures such as AAV vectors.
A preferable solution would be the adoption of membrane or fiber-based technologies. In these processes, ligands are bound to the surface of the structure, allowing for much higher flow rates to be used and improved binding and release kinetics. The development of these resins will depend on suppliers working with end users who have ready access to vectors for development. This will become increasingly important as processes scale up to 2,000 L-plus and the demand grows for increased throughput as more vectors are commercialized.
Removal Of Empty Capsids
The production of viral vectors inevitably results in the production of incomplete non-functional capsids, which are potentially immunogenic. Empty capsid levels can be as high as 90% in culture supernatants. While there are no specific guidelines on capsid ratios, empty capsids should be reduced to a minimum, and a full-capsid level minimum of 70% has been proposed.
The only reported routes to separate full and empty capsids are through density, using ultracentrifugation, and charge, via ion-exchange chromatography. Despite the challenges of scale-up and operator dependencies, ultracentrifugation separating on density differences is widely used, including in commercial processes, because of its robustness and simplicity.
Separation via ion-exchange exploits the small charge differences in full and empty capsids. It is more challenging, but it’s potentially a more scalable approach and less operator-dependent. Many producers are developing platforms around this approach. While some developers have been successful using it, ion-exchange is not universally applicable and outcomes appear to be serotype-dependent. Outcomes are also dependent on the initial levels of full capsids generated and the consistency of the levels. The real challenge with both approaches is the ability to scale and retain the required levels of separation and process yields.
In most ion-exchange processes, the separation is achieved using gradient elution procedures, which do not readily scale in terms of maintaining resolution and the need for pooling of fractions. There are also challenges with robustness. The process must be able to cope with variable levels of empty capsids in the feedstock. The long-term solution around the biology of vector production within the cells and vector design is to improve the production and assembly processes within the cell to give consistent and higher levels of complete capsid production.
Virus Filtration
The proposed ICH Guideline Q5A(R2) would require downstream processing of mammalian-sourced vectors to include at least two orthogonal virus removal steps, usually through low pH hold and viral filtration. To date, this has not been a requirement for viral vectors due to the technical challenges of performing these steps and the limited number of patients being treated with these products.
However, it has been recognized that AAV vectors are sufficiently robust to withstand exposure to low pH conditions, and their relatively small size of 25 nm means that it may be possible to remove larger virus particles through filtration without removal of the AAV particles. This requirement has not been enforced by regulators to date, but that may change as more patients are treated. The challenge will be material supply for these studies due to cost, and there will be a need for generic solutions, especially for early-phase products.
Drug Product
Product formulation includes critical steps that affect drug product delivery and stability. Limited work on formulation processes has been performed for AAV vectors in the past. Manufacturers simply relied on ultra-low temperature formulations for stability. But there is now an increasing body of work developing approaches for product stability and control during drug product manufacturing processes and product storage.
Conclusion
In summary, we are now seeing development at all stages of the manufacturing processes, both within producers and in partnerships with suppliers, technology providers, and academic groups.
Some of these developments, especially around the basic biology of vector production, present inevitable risks and will take time. Others are very much ongoing between vector producers and suppliers and will have more immediate effects. There are clearly challenges in the production of AAV vectors, but there is clear reason to believe that we will see significant improvements in vector production processes over the coming years.
About the Author:
Tony Hitchcock is the principal and owner of AGH Bioconsulting. He has spent his decades-long career in the in the biotechnology field, with over 30 years in the production of complex biologic for clinical trials in the European Union and U.S. He has worked in areas of process development and manufacturing with experience in engineering and process systems. He has worked on the development of more than 30 products for clinical trials including plasmid DNA, viral and bacteriophage products, and recombinant proteins from microbial, mammalian, and insect cell sources. Contact him at tony@aghbioconsulting.com.