Developing A Viral Gene Therapy Manufacturing Process
By Mark Haydock, biologics CMC consultant, Agile Biologics Consulting LLC
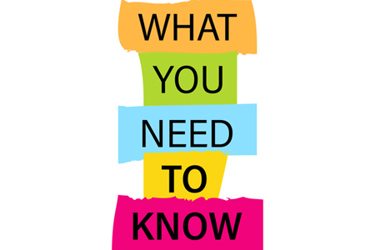
There are two main modes of viral vector production. The first is a transient production system in which a packaging cell line is transfected with the therapeutic gene along with additional genes that provide the viral helper function required for replication. In this system, the helper genes can be transfected through one or more plasmids or a helper virus.
The second viral vector manufacturing system uses a stable producing cell line. Stable producing cell lines allow for more scalable, simpler, reproducible, and cost-effective viral vector manufacturing processes. However, stable cell lines are difficult to develop and require substantial time and effort to generate.
Manufacturing viral vectors by transfection is flexible and efficient and does not require time consuming development of stable cell lines, significantly decreasing the time it takes to advance a new therapy from discovery to the clinic. However, transfection-based methods have disadvantages, such as the requirement for costly GMP-grade plasmids. Also, a consistent and reproducible transfection at GMP manufacturing scale can be tricky to implement and requires optimization. Maintaining batch-to-batch viral vector consistency is also a concern.
Currently, the majority of viral vectors are manufactured using transient production systems. This is because the flexibility, significant time savings, and convenience often outweigh the cost of GMP plasmids and the need for transfection optimization.
This article describes a transient transfection adeno-associated virus (AAV) manufacturing process that uses a triple plasmid transfection strategy into HEK293T cells, a human suspension culture cell line. The three transfected plasmids include the transfer plasmid, which contains the therapeutic gene, a helper plasmid that contains a set of genes from adenovirus that allow for AAV replication, and the AAV capsid/replication (cap/rep) plasmid that codes for three capsid proteins and a set of genes, that when translated, mediate AAV replication.
I choose this system because of the growing popularity of AAV for delivering in-vivo gene therapies, the preference of transient transfection systems by emerging biologics companies, and the increasing number of CDMOs offering this system as a platform process to simplify IND filings and significantly reduce CMC timelines.
The steps covered in this article include AAV vector design, plasmid production, culturing the packaging cell line, transfection of the packaging cell line, viral vector production, bioreactor harvest, viral vector purification, and final formulation. This article focuses on producing the transfer plasmid and viral vector and assumes that the CDMO developing and manufacturing this product keeps ready stocks of high-quality GMP helper and rep/cap plasmids on hand.
AAV Vector Design
The transfer plasmid is created by inserting the therapeutic gene of interest between the two internal terminal repeats (ITRs) of an empty AAV vector. Only the DNA sequence inserted between the ITRs gets packaged into the final AAV capsid. Anything outside the two ITRs is not packaged into the final AAV capsid.
The primary consideration when designing an AAV transfer plasmid is the packaging size of the expression cassette. An AAV single strand AAV(SAAV) product has a total packaging capacity of ~5.0 Kb, including the two ITRs. Since the two ITRs of AAV are about 0.2-0.3Kb total, the transgenic sequence that can be packaged into the virus must be smaller than ~4.7 Kb.
The vector elements that need to be optimized include the poly-A tail, regulatory elements, enhancers, and introns. In all of these cases, the primary consideration for designing AAV vectors is that the total transgenic DNA sequence packaged into each AAV capsid must be within the ~4.7 Kb packaging limit. This size limitation has largely prevented the use of large regulatory elements to control therapeutic gene expression with AAV vector systems. To better control the therapeutic gene expression, tissue-specific or temporal/regulatable promoters are sometimes used.
For coding sequences larger than ~4.7Kb, a longer therapeutic gene can be split between two separate viral vectors. When these viruses co-infect a cell, concatemers form that are spliced together so the full-length therapeutic gene can be expressed. Another technique for increasing packaging capacity depends on homologous recombination. This method divides a gene between two separately packaged transfer plasmids with substantial DNA sequence overlap. Co-expression of both viral vectors induces homologous recombination and expression of the full-length therapeutic gene.
Both methods allow therapeutic genes longer than 4.7 Kb to be expressed but are significantly less efficient than a single-stranded AAV vector. Developing more efficient processes to express therapeutic genes longer than 4.7Kb would dramatically increase the potential applications for AAV gene therapies.
The AAV serotype is a critical element of viral vector design because different AAV serotypes target different human tissues. Serotype AAV2 is the best characterized and most commonly used for research. Although AAV2 is the most popular serotype, due to tissue targeting, other serotypes can be more effective as gene delivery vectors. Examples include serotype AAV6 for infecting airway epithelial cells, AAV8 for hepatocytes, AAV1 and AAV5 for gene delivery to vascular endothelial cells, and AAV9 for neurodegenerative disease.
Process Development
To develop a robust and FDA-compliant drug substance manufacturing process, always use high-quality, well-characterized raw materials. Also try to only source from vendors who test and closely monitor their incoming raw materials and do not regularly change suppliers. Subtle changes in the raw materials used to produce the AAV vectors can increase batch-to-batch variability and lead to an inconsistent product. Also try to develop manufacturing processes that are animal component-free. If using animal-derived components during manufacturing is unavoidable, obtain certificates of origin and BSE/TSE-free statements to include in the IND.
For process development and GMP manufacturing, only use plasmid producing bacterial strains and AAV packaging cell lines with a well-established regulatory history. Since they already have clearly defined regulatory paths to qualifying their use for GMP manufacturing, this will significantly simplify the IND approval process.
For an AAV triple transfection process, the cell density, transfection volume, plasmid DNA concentrations, plasmid ratios, polyethylenimine (transfection reagent) to DNA ratio, incubation time of mix prior to transfection, temperature, the addition of supplements, and time of harvest all need to be optimized. Due to the number of factors that must be optimized, many CDMOs use DOE to optimize the transfections ratios for maximum quality and quantity of viral vectors.
The downstream viral vector purification process needs to incorporate dedicated viral clearance steps. Viral clearance studies are also required to show that the manufacturing process can clear any viruses that may have been introduced into the manufacturing process. Since the AAV virus has a diameter of 20-25nm, incorporating a viral filtration step with 35-50nm pore filters can be used for removing larger viruses. Heat and low pH also have minimal impact on AAV while inactivating many other viruses. Since AAV is a non-enveloped virus, detergents also can be used to inactivate enveloped viruses. However, Triton X-100, a popular detergent, degrades into estrogen-like compounds that may affect wildlife, making it no longer allowed for use in the European Union. If a detergent must be used, try incorporating another detergent, such as Tween 20, into the manufacturing process. Viral clearance for viral gene therapy products is more complicated than other biologic products because the product is a virus. ICH Q5A was recently revised, which should help clarify the viral clearance expectations for viral gene therapies.
To protect patients against potential adventitious agents in the product, a panel of safety tests must be performed to qualify all cell banks used for GMP manufacturing. Additional safety testing must also be performed on all plasmids, the viral vector bulk harvest, and the viral vector product. These testing requirements are described later in this article.
The combination of sourcing animal component-free raw materials, safety testing, and incorporating dedicated viral clearance steps into the manufacturing process, provides the best assurance of patient safety. During process development, it is vital to understand the risks associated with starting materials and the proposed manufacturing process and mitigate them early in development.
Once the plasmid and viral vector manufacturing process are developed, the final formulations must be chosen. A suitable formulation must be protective against any future stress conditions the final product is likely to encounter. Formulation development studies need to include elevated temperature conditions to justify any product manufacturing hold times or temperature excursions. As part of formulation development, always perform freeze-thaw studies with enough cycles incorporated to support the freeze-thaw of a reference standard for product release and stability testing. If the plasmids or viral vectors are stored as a frozen liquid, additional freeze-thaw cycles will be needed to support frozen storage.
When developing the manufacturing process, always choose scalable processes and equipment such as bioreactors instead of shake flasks and chromatography purification methods instead of ultracentrifugation. When possible, use established platform technologies that the regulatory agencies are already familiar with. This will make approval of an IND submission much simpler.
Transfer Plasmid Manufacturing
The transfer plasmid must be grown in a recombination-deficient bacterial system, with E. coli most often used. Using a recombinant-deficient bacterial strain helps prevent ITR loss during plasmid propagation with the complete loss of an ITR, eliminating the ability for final AAV vector packaging.
Large-scale GMP plasmid preparations are usually produced using a fed-batch process and harvested using centrifugation. Once harvested, the bacterial cells undergo alkaline lysis followed by clarification and chromatography to purify the plasmids. Various chromatography processes have been developed for plasmid purification, with anion exchange and hydrophobic interaction chromatography most often used.
Traditional protein chromatography resins are not recommended for purifying plasmids because the plasmid’s size usually causes diffusion limitations. This is because the resin pores are too small to allow the plasmids to interact effectively with the chromatography matrix. This has been addressed using monolith and membrane chromatography systems and adding extenders to chromatography resins to improve interactions between the plasmids and the chromatography matrix.
After the plasmids are purified, they are usually formulated into the final storage buffer and concentration using tangential flow filtration (TFF). The plasmids are then tested to confirm their identity, form, purity, and safety. This is done by employing various methods such as UV spectroscopy, agarose gel electrophoresis, DNA sequencing, restriction enzyme mapping, SDS-PAGE, micro-BCA, ELISA, qPCR, residual antibiotic analysis, bioburden, mycoplasma, pH, appearance, and endotoxin testing.
Limits for bacterial host cell contaminant levels, including DNA, RNA, and protein, must be <1%. The amount of endotoxin in plasmid DNA vaccine preparations should not exceed 40 EU/mg plasmid. The topology of the plasmid (% supercoiled) must also be confirmed with a minimum regulatory requirement of >80% supercoiled plasmid content.
Transfer Plasmid Master Cell Bank
To support GMP manufacturing, a master cell bank (MCB) needs to be created and qualified. This cell bank must be derived from a single well-isolated bacterial colony. In addition, the identity, purity, plasmid presence and identity, therapeutic gene expression, and safety must be confirmed. Plasmid presence and identity can be confirmed by bacterial growth on selective medium, restriction enzyme digest, and full DNA sequencing. The identity of the bacterial strain also needs to be confirmed with gram stain analysis and analytical profile index (API) or 16S rRNA ribosomal sequencing. The purity of the MCB must be confirmed by testing a minimum of 10 random colonies that show no inappropriate organisms and are negative for bacteriophage. Sterility, endotoxin, bacterial and fungalstasis, and mycoplasma testing are also required.
Upstream Viral Vector Manufacturing
The example in this article uses HEK293T, a human suspension packaging cell line. Suspension cell lines are better for scaleup, but some AAV products get poor yields with suspension culture. HEK293, an adherent cell line, is an option in this case. Adherent cells do not require daily cell counts but require scaling out instead of up. For an adherent process, use animal-free trypsin.
For the transient triple plasmid transfection process, the HEK293T cells will be grown, expanded, and then transfected with the AAV transfer, cap/rep, and helper plasmids. The culture continues while the viral vector is produced and packaged into AAV particles.
Once the bioreactor is harvested, the HEK293T cells undergo chemical cell lysis. Freeze-thaw is also possible for smaller development batches, but for larger-scale manufacturing, batch volumes quickly become an issue. DNA fragmentation is then performed on the lysed cells with a nuclease such as benzonase. The cell lysate is then clarified using depth filtration or centrifugation. If depth filtration is used, it is best to use PES membranes since AAV particles may stick to other filter surfaces, reducing the final yield. This unprocessed bioreactor harvest material must be tested for adventitious agents, identity, titer, AAV replication competence, transmission electron microscopy, bioburden/sterility, and mycoplasma.
The clarified harvest is then concentrated, and buffer exchanged by tangential flow filtration (TFF) to prepare for downstream processing. When manufacturing AAV therapies, a TFF membrane with a molecular weight cutoff of 300,000 KDa is more efficient for removing host DNA and protein than a 100,000 KDa membrane.
Downstream Viral Vector Manufacturing
The AAV vectors are then purified. Small-scale research and development batches can be purified using ultracentrifugation, but larger manufacturing batches must be purified using chromatography due to size limitations. Different AAV serotypes behave differently during chromatography, so creating a platform downstream manufacturing process that works across all the different AAV serotypes is difficult.
Affinity purification is often employed as a first bulk cleanup step to remove host proteins and DNA. AAV serotype specific affinity resins are now available for a growing number of serotypes. Some affinity resins are NaOH-regeneratable.
A mix of capsids with and without a DNA payload is generated during upstream viral vector manufacturing. The particles with DNA are referred to as full capsids, and the ones without DNA are empty. While the impact of empty or partially filled capsids is not fully understood, regulatory agencies consider them a contaminant that must be removed.
Removal of these empty capsids was initially done using ultracentrifugation but, due to batch size processing limitations, is being replaced by chromatography for larger-scale GMP manufacturing. Since the DNA in full capsids is negatively charged, anion exchange is commonly used to remove the empty capsids. In addition, anion exchange chromatography also removes any HCP and DNA that were not removed during the affinity purification step.
As with plasmid production, the traditional chromatography resins used for protein purification should not be used for virus vector purification because of diffusion limitations. These limitations are also being addressed with monolith and membrane systems and adding extenders to chromatography resins to improve interactions between the viral vectors and the chromatography matrix.
For large-scale GMP manufacturing, TFF is usually used for the final formulation step. The final formulation helps with the stability of the viral vector product but also can enhance transduction efficiency in-vivo. Once the viral vectors are formulated, they are QC-tested to confirm the product’s genetic and protein identity, physical and functional viral titer, potency, AAV capsid content (empty-to-full ratio), process-related impurities such as residual benzonase, host cell-related protein, and DNA/RNA impurities, as well as safety through sterility, endotoxin, mycoplasma, replication competence, and adventitious agents testing.
Packaging Line Master Cell Bank
To support GMP manufacturing, a MCB of the HEK293T packaging cell line needs to be created and qualified. This MCB must be derived from a single clonal cell line. This cell line also needs to be genetically stable. The origin, history, cell line morphology, and growth characteristics must all be considered. The cell line's identity must also be confirmed through methods such as isoenzyme analysis or CO1 barcode assay.
To ensure patient safety, the HEK293T MCB must be tested for sterility, mycoplasma, bacterial and fungalstasis, retroviruses, inapparent viruses, and virus contaminants. Since this cell line will produce AAV vectors, it must also be tested for replication competent retrovirus. The HEK293T MCB also must be tested for specific human viruses that include EBV, HBV, HCV, CMV, HIV 1 and 2, AAV, B19, and HTLV 1 and 2. Because of the HEK293T cell line history, testing for bovine and porcine viruses is usually performed. Depending on the history of the particular packaging cell line, testing for canine and simian viruses may also be required.
Conclusion
This article describes a transient transfection AAV manufacturing process and provides a high-level overview of the steps required to develop and manufacture DNA plasmids and viral vectors. This article focused on establishing a controlled manufacturing process while highlighting recent advancements in DNA plasmid and viral vector manufacturing technology. This article also covered the required manufacturing safeguards needed to ensure patient safety during clinical trials. Please contact the author with any questions.
An earlier version of this article was published on the Agile Biologics Consulting LLC website. Republished with permission.
About The Author:
Mark Haydock is a biopharmaceutical CMC consultant and founder of Agile Biologics Consulting LLC. Before becoming a consultant, he served as vice president of CMC at Cue Biopharma. He has more than 30 years of experience in biopharmaceutical CMC operations, at Chiron, Aventis, Medarex, Morphotek, Eisai, and Mersana Therapeutics. During his career, Haydock established the CMC departments for three successful VC-funded companies and has supported over 30 products. He holds a B.S. in microbiology from California Polytechnic State University and an M.S. in molecular genetics and cell biology from the University of Chicago.