3D Printing For Pharmaceuticals & Medical Devices: A Primer With Risk Considerations
By Tim Sandle, Ph.D.
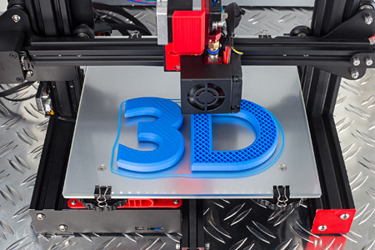
Over the past decade, implementation of single-use technologies for pharmaceutical and medical device manufacturing has introduced advantages in terms of design, sterility assurance, and a reduction in energy usage, relating to areas like sterilization and cleaning. Within pharmaceuticals, these technologies introduce a new way of thinking in terms of the design space and process flows.1 Types of single-use technologies relevant to aseptic processing include tubing, capsule filters, single-use ion exchange membrane chromatography devices, single-use mixers and bioreactors, product holding sterile bags in place of stainless-steel vessels (sterile fluid containment bags), connection devices, and sampling receptacles.2 With medical devices, more niche products tailored to the requirements of individual patients can be produced at a lower cost and with improved compatibility.3 Interest in such applications increased from 2015, when the FDA approved the first 3D printed product. This shift in design and production has been facilitated by the advent and improvement of 3D printing.
Since then, advances in 3D printing have led to novel developments in biomedical science, such as the 3D printing of thick, vascularized, and perfusable cardiac patches to serve as functioning hearts.4 Areas of application in pharmaceuticals include drug delivery systems, such as controlled release and microneedles; pharmacy dispensing aids and drug-eluting devices, including for patients who require special personalized medications for long-term care; and single-use disposable technologies.
With medical devices, innovations have been faster as it is an ideal technology for fabrication of parts in industries that typically do not operate in economies of scale, such as medical implants. Three example innovations that fall within “medical devices” include: printing cranial composites of polylactic acid plastics that differentiate MS tumors from cancerous or false positives; open-source prosthetics; and assisting with jaw replacement surgery by printing skull renderings made of plastics designed to the precise measurements of the jawbone. With each, 3D printing can deliver precise measurements to medical production facilities, saving time critical to patient prognosis.
What Is The 3D Printing Process?
3D printing (or additive manufacturing, as opposed to subtractive processes in which material is cut, drilled, milled, or machined off), is the process by which digital 3D design data (as a computer model) is used to build up a physical structure, where the component is formed in layers by the deposition of material.5 The materials can be plastics, composites, or biomaterials and these range in shape, size, rigidity, and color. More recently, printing of hollow systems intended to convey different drug formulations has expanded the versatility of 3D printed applications. Drug distribution can be assessed using fluorescent dye to visualize the product path through filaments and implants. The steps required for 3D printing are straightforward, as per the commonly used fused deposition modeling method:
- The first step is melt-extrusion at the required processing temperature (generally, above 100°C).
- The second step subjects the extruded cooled filaments to heating and melting during printing through a nozzle at even higher temperatures.
An alternative method is stereolithography, in which an ultraviolet light is shined into a vat of ultraviolet-sensitive photopolymer, tracing the object to be created on its surface.
The complexities are with the design concept, computer programming (which may include algorithm development), and material selection. With the latter, certain risks are presented, and these are examined below.
Risk Considerations
The key risk elements include material degradation and an alteration to the mechanical properties of the plastic, which could cause polymers to bend under the stress of the additive manufacturing process. These risks require a review of appropriate materials, assigning critical attributes, and a controlled material purchase and release process. Additives may be required to avoid desired materials becoming brittle. Desired materials include plasticizers or solubilizers (designed to foster flexibility). As part of quality-by-design and later quality control assessments, the following need to be considered:6
- The appearance of the fabricated item, such as discoloration
- Tackiness
- Flexibility, where the item can be subjected to different forms of tensile testing
- Mechanical strength
To fully understand the above hazards, you need to understand and document the risks identified for material supply and for each step of the manufacturing process, as well as mitigations of these risks. Assess these risks both post-printing and following any sterilization process, given the stress that sterilization technologies impart. It is important to continue with assessments across the shelf life of the product. An understanding of the intended use of each individual device must frame the overall consideration of risk. Later on, you must identify and assess any changes to the device, manufacturing process, or process deviations for any potential risks that might be introduced.
A further risk that you need to assess with both the core material and the use of any additives, catalysts, binding and curing agents, uncured monomers, and plasticizers is toxicology. This includes the risk posed by leachable impurities. This needs to be extended to consider any risk of residual manufacturing material, such as excess starting material or support material, remaining on the finished device. To assess these risks, careful consideration of materials and the reaction of materials in the presence of the product is required as part of a wide assessment into biocompatibility. These considerations must be framed by the risk profile in relation to the intended user of the material (for a medical device) or in relation to a specific product, when used in pharmaceutical processing.
Limitations
While 3D printing promises many innovations, at present there are some limitations. Restrictions include:
- Limited materials, since the current selection of raw materials is not exhaustive
- Restricted build size relating to the types of 3D printers available
- Post-processing, which is limited by the extent that fabricated materials can be fashioned
- The effect of sterilization methods
- Large volumes
- Part structure
- Design inaccuracies
- Regulatory approval steps
The Future Is Bright For 3D Printing In Pharmaceuticals & Medical Devices
Greater advances have been made with single-use systems than with drug delivery.7 However, the types of limitations listed above are not, in the longer term, insurmountable as technology and understanding progresses. As with any novel technology, there continues to be, at times, uneven progress. Overall, though, as an area of advanced automation and design, the application of 3D printing is only set to increase.
References
- Hodgkinson, M. (2014) Enhancing Microbial Control and Sterility Assurance in Aseptic Processing by Implementing Single-Use Technology, American Pharmaceutical Review, at: https://www.americanpharmaceuticalreview.com/Featured-Articles/158936-Enhancing-Microbial-Control-and-Sterility-Assurance-in-Aseptic-Processing-by-Implementing-Single-Use-Technology/
- Sandle, T. (2017) Establishing a Contamination Control Strategy for Aseptic Processing, American Pharmaceutical Review, at: https://www.americanpharmaceuticalreview.com/Featured-Articles/335458-Establishing-a-Contamination-Control-Strategy-for-Aseptic-Processing/
- Sandle, T. (2017) Read How 3D Printing Can Save Your Life, Open Health News, at: https://www.openhealthnews.com/news-clipping/2017-06-30/read-how-3d-printing-can-save-your-life
- Sandle, T. (2018) Tiny 3D-printed heart fabricated complete with blood vessels, DX Journal, at: https://dxjournal.co/2019/04/tiny-3d-printed-heart-fabricated-complete-with-blood-vessels/
- Bucchi M. Saracino B. (2016) Visual Science Literacy: images and public understanding of science in the digital age. Sci Commun. 38: 812-819
- Alhijjaj, M., Belton, P., and Qi, S. (2016) An investigation into the use of polymer blends to improve the printability of and regulate drug release from pharmaceutical solid dispersions prepared via fused deposition modeling (FDM) 3D printing, Eur. J. Pharm. Biopharm., 108: 111-125
- Seoane-Viaño, I., Trenfield, S., Basit, A., Alvaro Goyanes, A. (2021) Translating 3D printed pharmaceuticals: From hype to real-world clinical applications, Advanced Drug Delivery Reviews, 174: 553-575
About The Author:
Tim Sandle, Ph.D., is a pharmaceutical professional with wide experience in microbiology and quality assurance. He is the author of more than 30 books relating to pharmaceuticals, healthcare, and life sciences, as well as over 170 peer-reviewed papers and some 500 technical articles. Sandle has presented at over 200 events and he currently works at Bio Products Laboratory Ltd. (BPL), and he is a visiting professor at the University of Manchester and University College London, as well as a consultant to the pharmaceutical industry. Visit his microbiology website at https://www.pharmamicroresources.com.