Comparing Alternative Viral Vector Purification Tech
By Jack Vicalvi, principal scientist, J2 Biopharm Associates LLC
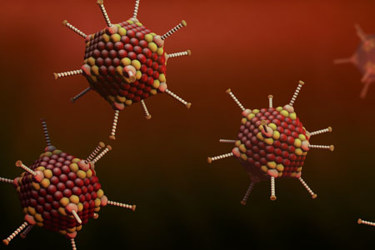
This is an overview of selected innovative products and approaches developed during the past 15 years that have contributed to enhancements allowing manufacture of viral vector therapeutics with higher recoveries and purities.
This is the second part of a series looking at some specific purification technology advances. The first part discussed downstream equipment and processes for monoclonal antibodies.
In part two, we examine how these products can change the way we manufacture such viral vector therapeutics, where they can be used in current process development schemes, and what advantages they confer over previous methods. We touch upon the situational economics that drive the use of these innovative products or approaches. Finally, we focus on GMP scale-up models for practical purposes: if it doesn’t scale at GMP, it doesn’t matter how well it works in development.
Viral Vector Process Flow Diagrams
Viral Vector Harvest Clarification
Clarification of viral vector harvests is more complicated than mAb clarification. Traditional clarification usually begins with differential or gradient centrifugation. This can be problematic at best for GMP manufacturing at scales greater than 100 L, since centrifuge bucket batch load size is generally small (10 to 20 L); then there’s aerosol generation, cleaning, and validation concerns, as well as capital equipment costs that may be show-stoppers. Even with a centrifugation step, depth filtration and sterile filtration remain necessary. The use of charged depth filters may be untenable depending on the virus since most viruses are bound by anionic charge moieties. However, in the presence of 200 to 300 mM NaCl, many viruses will not be removed by such devices, whereas some HCP and host cell DNA is still bound.
Enveloped viruses such as lentiviruses and alphaviruses are more labile and may not fare well with depth filtration. However, the alphaviruses are more robust and may be amenable to normal, and even charged, depth filtration. In these cases, we use the Pall SupraCap 100 (4 to 2.1 µm), Pall HCDII (1.2 µm), and AcroPak 500 (0.8 to 0.45 µm) in series. The Millipore DOHC, COHC, and XOHC membranes may also be used. Use of 0.2 µm filters usually results in significant losses and should be avoided until final filtration. Maintaining an aseptic closed system during processing is imperative.
The more popular non-enveloped viruses such as adenoviruses (AV) and adeno-associated viruses (AAV) may be clarified using the 3M charged depth filters in the presence of 180 to 250 mM NaCl. We use the 05SP (10 to 2 µm) and the 60SP (3 to 0.2 µm) filters in series for non-enveloped and some enveloped alphaviruses.
Viral Vector Capture Step
The traditional method for viral vector capture is AIEX. This may be accomplished using resins or membranes. For enveloped viruses, the membrane approach is usually best since it is much gentler on the virus than the torturous path through a packed column. The most common devices employed include the Pall Mustang Q, Sartorius Sartobind Q-STIC, and the BIA CIMultus monolith. The greatest drawback with membranes or monoliths is their low capacity, resulting in the need to use multiple cycles. However, using a column with a short bed height (6 to 10 cm) may work as well, with the added benefit of significantly increased capacity over membranes. Commonly used non-enveloped viruses may be captured either by resin or membrane devices.
An alternative method is using affinity chromatography. Many viruses have heparin-binding sites on their surfaces, including enveloped viruses. We have had success with Heparin HyperD as a capture step for an alphavirus following M-SAN nuclease digestion and clarification, resulting in >90% recovery with excellent removal of hcDNA and significant reduction of HCP while running the column at 450 cm/hr. The HyperD ceramic resins are operationally similar to polystyrene resins. There are other purification protocols that have incorporated Cellufine Sulfate or Capto DeVirS as an initial capture step; these resins have worked well in the purification of flaviviruses.
Table 1. Viral Vector Capture Comparison (AIEX) of Soft vs. Rigid Resins vs. Membranes
*Q Sepharose @ $6,000/L = $60,000/column x 2 (GMP back up) = $120,000 + columns ($40,000-$60,000 each).
**POROS HQ @ $7,500/L = $75,000/column x 2 (GMP back up) = $150,000 + columns ($40,000-$60,000 each).
***Mustang Q XT @ $18,000 ea x 2 (GMP back up) = $36,000.
Primary Polishing Step
In many traditional approaches for non-enveloped viruses there may not be any polishing steps. However, it might be advisable to have something in place to remove some of the remaining HCP. Options may include CIEX, HIC, SEC, or mixed modal. Mixed modal may incorporate all three, depending on the pore size of the resin selected. If CIEX is selected, be aware that a pH near or below the PI of the virus may inactivate it; you will recover a lot of virus, but it will not be infective. Capto Core 700 has an anionic moiety on a long hydrophobic arm attached to agarose with pore sizes that exclude viral diffusion. In the presence of salt (e.g., from a capture elution step) the virus is not bound to the AIEX; however, hcDNA is bound and HCP is bound by the hydrophobic moiety. Capto MMC has a cationic moiety on a long hydrophobic arm attached to cross-linked agarose that may be used in a flow-through operation.
In contrast, enveloped viruses do not behave well with HIC-based resins; their membranes are very hydrophobic and do not easily release under normal operating conditions. Most enveloped viruses also have a tendency to bind to many CIEX resins under normal operating conditions. An alternative approach is to employ an AIEX resin or membrane after affinity capture as the primary polishing step.
The SEC approach is another alternative, allowing the virus to pass through in the exclusion volume while trapping HCP and smaller contaminating fragments in the matrix. BioWorks has an SEC resin that operates well at high capacity (20% of resin volume) and reasonable velocity for such resins (120 to 180 cm/hr), whereas most SEC resins operate best with a load of 3%-10% of resin volume and a linear velocity of 20 to 60 cm/hr.
Secondary Polishing Step
In both traditional and alternative methods this step is generally omitted. However, if the HCP levels or empty/full capsid ratios are still higher than desired after following the capture and mixed modal polishing steps, an additional polishing step with an SEC resin may be appropriate as described above. Alternatively, a recently developed scalable automated rotary ultracentrifuge from Alfa Wasserman may be useful at this step for removal of host cell contaminants, as well as empty or partially filled capsids.
Ultrafiltration/Diafiltration (Tangential Flow Filtration) Step
Many methods try to use the TFF step as a means of reducing HCP and hcDNA. Generally, this seldom works since these contaminants, if still present, are likely associated with the target molecule either by ionic or hydrophobic interactions. In addition, tight membranes of 10 to 30 kD simply do not have pore sizes that are large enough to allow most HCPs (30 to 60 kD) to pass through. While most viruses are much larger than 300 kD, they are malleable and can squeeze through these membranes under normal operating conditions (10 to 15 psi). Under most circumstances, the 100 kD membranes work best, although AAVs may require a 50 to 70 kD membrane for maximum recovery.
Final Thoughts On Columns, Resins, And Membranes
The main difference between mAb purification and virus purification is viral clearance. The methods used for viral clearance in mAb processes (other than filtration) are the methods used for virus purification. The chromatography principles remain the same: utilizing differences in charge (due to pH and conductivity), molecular weight, hydrophobicity, and maybe ligand affinity.
Clarification is similar as well, since the harvest is loaded with proteolytic enzymes and cell debris just as in mAb production, and these contaminants must be removed as early as practicable. The important thing is to start the process with your best chance to not only capture your target molecule but to remove as many contaminants as possible.
Choosing columns, resins, and membranes for viral vector purification raises the same concerns as with mAb purification. Repligen offers Opus prepacked disposable columns ranging from 50 µL up to 150 L (80 cm diameter). They can be packed with more than 300 user-specified resins in a range of bed heights from 0.25 to 30 cm. Alternatively, Sepragen Radial Flow Superflo columns operate four to 10 times faster at a lower operating pressure with lower pool volumes than conventional axial columns, allowing for significant reduction in purification costs. These columns also are available in a prepacked format with any resin. The interesting thing about radial flow columns is the bed height is usually 5 to 7 cm and scale-up is achieved by increasing the tube length, similar to a hollow fiber approach. This arrangement could be more beneficial for labile enveloped virus purification.
Rigid resins may be operated at high linear velocities (800 to 1,200 cm/hr) without significant loss of productivity. Alternatively, Pall Danaher is commercializing a fiber-based chromatography platform obtained via the acquisition of Puridify (from Cytiva). The fiber format has an open pore structure that allows for convective mass transport, leading to flow rate-independent binding capacities and cycle times of minutes compared to hours for traditional resin chromatography. These membranes and fibers can be used for rapid cycling across a single batch before disposal, and the larger surface areas and microporous structures may also make these fiber technologies suitable for the purification of viral vectors.
With non-enveloped viruses, capture by AIEX resin is the most popular approach. However, an affinity resin allows for greater reduction of HCP and hcDNA as flow-through, whereas AIEX will bind HCP and hcDNA. For AIEX, selective buffer washes may be used to reduce some HCP and remove empty capsids prior to elution; hcDNA tends to bind more tightly than most HCP, requiring higher salt and/or NaOH washes for removal from the resin. As mentioned previously, the choice of matrix composition also contributes to either removal or retention of contaminants and may either eliminate or increase the need for further purification downstream of any step.
As with mAbs, the order following the capture step for viral vectors should be dictated by the elution conditions from the previous step. Reducing manipulation of buffers between steps avoids unnecessary TFF steps. Some simple buffer dilutions may be required to alter the conductivity or pH of the previous elution step and should be handled in mixing tanks when possible, especially for bind-elute steps. Flow-through steps are usually reserved for the last step prior to TFF since they tend to increase the volume of the drug substance. Using membranes versus resins in flow-through steps helps to reduce the resulting increase in volume and shorten the TFF concentration step.
Choosing the appropriate molecular cutoff for the TFF membrane is critical. Viral vectors are more variable in size than mAbs; however, they are always much larger than mAbs, and they also may change shape under pressure and squeeze through pores greater than 100 kD. We have found enveloped alphavirus particles (90 to 100 nm) in the diafiltrate from 300 kD membranes under normal operating conditions (10 to 15 psi), but not in diafiltrate from 100 kD membranes. Smaller viruses, such as AAV, will require tighter pore sizes (30 to 70 kD), making HCP removal earlier in the process more imperative.
Lastly, the key drivers in any purification strategy are cost and productivity. Always consider the manufacturing costs when designing your method. Relatively small increases in cost during process development may result in greater productivity and cost savings during manufacturing when chosen wisely. Whenever selecting a particular step (resin or membrane), always consider the GMP manufacturing cost (validation, suite time, buffer consumption, etc.). Also, let your critical quality attributes drive the process development; going the easiest or most inexpensive way early on may come back to haunt you in manufacturing.
References:
BioPlan Associates, Inc. (2019) Top 15 Trends in Biopharmaceutical Manufacturing, in 16th Annual Report and Summary of Biopharmaceutical Manufacturing Capacity and Production, Oct. 2019.
Challener, CA (2019) Seeking Solutions for Large-Scale GMP Viral Vector Manufacturing, Pharmaceutical Technology, Vol. 43:9.
GE Healthcare Life Sciences (2019) Intensified Chromatography Strategies, retrieved from https://www.gelifesciences.com/en/us/solutions/bioprocessing/knowledge-center/intensified-chromatography-strategies.
Lavelle, L (2019) What’s New in Manufacturing: Process Chromatography, BioPharm International, Vol. 32:9.
Masri, F et al. (2019) Viral vector manufacturing: how to address current and future demands?, Cell & Gene Therapy Insights 2019; 5(Suppl. 5), 949–970.
Moss, D (2019) Vector purification: issues and challenges with currently available technologies, Cell & Gene Therapy Insights 2019; 5(9), 1125–1132.
Pall Biotech (2019) Meeting the Challenge of Gene Therapy Manufacturing, GEN Vol.39:9.
Peters, RC (2020) Faster, Better Bioprocessing in 2020, BioPharm International, Vol.33:1.
Stevenson, RL (2013) Second-Generation Expanded Bed Adsorption Scales from Lab to Plant, American laboratory 45(2), February 2013.
Vicalvi (2020) “Suppliers' Contributions to Bioprocessing: Review of Who Developed Genuinely Significant New Technologies and Products” in 2020 17th Annual Report and Survey of Biopharmaceutical Manufacturing Capacity and Production, pp. 64-79.
About the Author
Jack Vicalvi is a principal scientist and director of downstream process development at J2 Biopharm Associates, a consulting firm, where he advises biopharmaceutical companies on GMP manufacturing processes and provides support for regulatory submissions. Before that, he worked for Pall Life Sciences as a principal scientist and downstream process development manager. Previously, his posts included leadership and research positions at Goodwin Biotechnology, Xcellerex, Exact Labs, Catalent, and Sunovion Pharmaceuticals. His undergraduate study was at St. Anselm College followed by graduate programs in immunology at Southern Illinois University and parasitology at Vanderbilt. Connect with him on LinkedIn.