Can ICH Q14 Ease Regulatory Risks Around Impurity And Potency Testing?
By Laura I. Salazar-Fontana, Ph.D., and Daniela Drago, Ph.D., RAC, NDA Partners
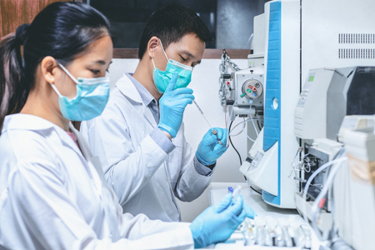
Robust and reliable analytical procedures are critical in the life sciences industry. In today’s complex regulatory landscape, where major treatments are developed and approved across different countries, changing an analytical procedure can be cumbersome and costly.
Over the years, pharmaceutical quality by design (QbD) has evolved with the issuance of ICH Q8 (R2)1, ICH Q92, and ICH Q103. The QbD principles together with a risk-based approach have facilitated the incorporation of process understanding into a control strategy that is based on sound science and quality risk management to ensure product quality consistency.
Changes in biotherapeutics product quality attributes (QA) — product meaning here both drug substance (DS) and drug product (DP) — are inherent to the complexity of their manufacturing process. Although a certain level of variability is acceptable, the goal is that a possible drift in process performance is quickly noticed by a well-defined quality system based on good process knowledge. Drifting in product quality also can be a consequence of changes in the performance of analytical methods used for quality control (QC) testing because of variations in essential procedure parameters. Acknowledging this, the ICH Guideline Q14 Analytical Procedure Development4 has been developed to extend the implementation of QbD tools (i.e., design of experiments (DoE)) to the life cycle management of these methods. The term "design of experiments" refers to a systematic procedure used to determine the relationship between factors affecting a process and the output of that process. By simultaneously examining multiple method parameters, system suitability testing, and controlling critical reagents, developers can efficiently maintain QC method performance through drug development. Specifically, this can be achieved by predefining an analytical target profile (ATP), analogous to a quality target product profile (QTPP), that warrants good life cycle method management by linking the anticipated performance criteria of the analytical measurement to the method’s intended purpose.
Global pharmaceutical companies fear facing significant delays and additional costs when an essential analytical method fails, especially for methods used to control critical quality attributes (CQA) such as purity and potency.
The adoption of ICH Q14 can help avoid these regulatory hurdles, ensuring reduced compliance risks and faster market access.
Ensuring Safe, Consistent, And Reproducible Therapeutics
A high-quality product is defined as one free of contamination and capable of consistently delivering the therapeutic action demonstrated by the clinical evidence described on its label.5 Adopting a QbD approach, as endorsed by the FDA as far back as 2009, means relying on scientific knowledge for product development rather than extensive testing. It is equally clear that good quality control relies not only on good manufacturing process knowledge but also on the proper selection and validation of the analytical procedures selected to monitor product QA, especially those linked to their therapeutic effect and safety.
The analytical methods used for release and stability testing need to be “fit for their intended purpose” as enunciated in ICH Q2(R2).6 This means that the chosen technology and its execution should reliably measure the particular attribute(s) with specificity/selectivity, accuracy, and precision at all stages of drug development. However, despite the rigor in selecting and validating analytical procedures, deficiencies still arise from inadequate development, such as suboptimal testing of critical reagents or selection of unsuitable operational parameter ranges.
From a quality perspective, a biologic must demonstrate continued safety, purity, and potency of the final product.7-8 Synthetic and recombinant peptides, albeit being classified as small molecules, must demonstrate the same attributes. Notably, the principles outlined in ICH Q14, which provide a systematic approach to the development and life cycle management of analytical methods, apply both to chemical (e.g., synthetic and recombinant peptides) and biological/biotechnological products.
This column will focus on how implementation of ICH Q14 can alleviate regulatory hurdles frequently linked to purity and potency methods.
Purity Testing Deficiencies
Purity means relative freedom from extraneous matter in the finished product, whether or not harmful to the recipient or deleterious to the product, except that which is unavoidable in the manufacturing process described in the approved biologics license application.7-8 Defining acceptable ranges for residual amounts of impurities requires solid knowledge and development of both the manufacturing process and the analytical procedures used to evaluate them.
Process-related impurities include residual host cell protein/DNA (i.e., residual components of the expression cell line), residual raw materials (i.e., culture media components, amino acids), or residual chromatography columns components (i.e., Protein A) used in the purification of recombinant proteins. While product-related impurities usually include degradation, clipped forms and/or different post-translational versions of the original molecule. In general, process-related impurities might usually pose potential safety risks, while product variants might, as well, modify the potency of the administered drug because they may still retain part of the biological activity of the intact protein/peptide. It is recognized that manufacturing changes can alter the impurity profile of a product and, therefore, purity needs to be closely monitored and controlled.
Chromatographic and electrophoretic methods are extensively used for this assessment. A large number of regulatory deficiencies are related to the performance of these methods.9 For example, some of FDA’s most commonly identified inadequacies are the use of a limit of quantitation (LoQ) sample outside the signal to noise (S/N) specification of the validated chromatographic method or the lack of consistency of product relative retention time (RRT) profiles, which make it difficult to define acceptable criteria ranges for consistent batch-to-batch purity.9 An example of another common deficiency in late development is the lack of method validation using a positive control that is representative of the final manufacturing process, which may compromise method selectivity. These types of deficiencies can be easily addressed by adopting a phase appropriate enhanced approach to method development. Per ICH Q14, the enhanced approach offers a “systematic way of developing and refining knowledge of an analytical procedure and demonstrating procedure understanding. Product and process understanding informs the quality attributes to be tested. The anticipated performance criteria for relevant performance characteristics should be documented in an analytical target profile.”
Potency Testing Deficiencies
Deficiencies in potency methods are critical from a regulatory perspective because they can result, in the worst-case scenario, in an approvability issue. Potency is interpreted to mean the specific ability or capacity of the product, as indicated by appropriate laboratory tests or by adequately controlled clinical data obtained through the administration of the product in the manner intended, to effect a given result.7 Testing can consist of in vitro or in vivo tests or a combination of both specifically designed for each product.8
Some of the most frequently reported regulatory deficiencies in potency methods are:
- selection of a method that is not representative of the drug’s mechanism of action (MoA),
- limited dynamic range due to interference of factors in the main signal readout, and
- highly variable cellular response due to poor characterization of the optimal number of indicator cells to be used in the assay.
It is recognized that defining the MoA of a product may sometimes be complex due to the involvement of more than one biological target; it therefore requires careful evaluation of multiple methods and signals to define quantitative limits around each variant with biological activity. A particular example of challenging products is cell-derived and genetically-modified cellular products. In this case, good characterization studies are crucial to define the biologically active components and orient the selection of the best analytical methods for their quantitation.
Some of these methods may be particularly sensitive to variations introduced by changes of critical reagent lots (e.g., number of passages of the indicator cell line) or instrumentation (e.g., replacement of an old luminometer by a new one). Kunzelmann et al. have recently published an applicable use of the DoE approach to manage the variability of the dose-response curve of a biologic’s potency assay 10 showing that simultaneous evaluation of multiple method parameters might be more informative than extensive testing of individual ones.
A successful example of integrating manufacturing and analytical method development QbD approach is seen in the optimization of lipid nanoparticle (LNP) formulations for RNA delivery (RNA-LNPs). Many critical formulation parameters can affect, among other CQAs, the potency of these nano-formulations.11 Thus, combining the systematic drug development approach (QbD) and the multivariate design and statistical analysis (DOE) to manufacturing with quality method development, can be very advantageous to ensure robust and reproducible product quality.
Conclusion
As of March of 2024, FDA has fully adopted ICH Q14. This guideline not only describes the scientific principles for development, change management, and submission requirements of analytical procedures, but it introduces the choice to implement an enhanced approach to analytical method development. This is not a new concept to quality since it is already applied to the evaluation of the manufacturing process. The advantage of extending this approach to the QC method performance is the ability to define a systematic way of quickly identifying a change in performance when it happens so a root-cause analysis and a mitigation plan can be put in place to promptly address a potential drift in method performance.
These principles can help not only to address some of the analytical limitations described early on for impurity and potency testing but facilitate early identification of interrelated method parameters through definition of a design space. Additional benefits of ICH Q14 are harmonization of scientific approaches and terminology (including multivariate analytical procedures and RTRT), improved analytical procedure knowledge, and risk management for continual performance improvement.
A potential hurdle in implementing ICH Q14 and the concept of an analytical QbD (AQbD) may reside in the inadequate use of DoE approaches. This can result in a design space that does not adequately reflect key parameters and ranges. However, proper training and engagement of experts can help overcome such a barrier. Another potential drawback may have its foundation in poor knowledge of the physicochemical and biological characteristics of the product because this can:
- hinder the selection of the suitable technology,
- mislead method development, or
- compromise the completeness of the ATP due to insufficient method performance data collection, making it difficult to discriminate between product and analytical drift.
Finally, combining key aspects of method development into the method validation documentation can greatly facilitate understanding of method performance. Increasing the clarity and completeness of the documentation is bound to reduce the number of regulatory queries and facilitate method transfer between testing laboratories.
Overall, extending a science- and risk-based approach to analytical method development helps harmonize principles for consistent product quality to ensure safe and efficacious therapeutic products.
References:
- FDA, Guidance for Industry, Q8(R2) Pharmaceutical Development, November 2009, ICH https://www.fda.gov/media/71535/download
- FDA, Guidance for Industry Q9(R1), Quality Risk Management, May 2023, ICH Quality https://www.fda.gov/media/167721/download
- FDA, Guidance for Industry Q10, Pharmaceutical Quality System, April 2009, ICH Quality https://www.fda.gov/media/71553/download
- FDA, Guidance for Industry, Q14 Analytical Procedure Development, March 2024, ICH-Quality, https://www.fda.gov/media/161202/download
- Yu LX, Amidon G, Khan MA, Hoag SW, Polli J, Raju GK, Woodcock J. Understanding pharmaceutical quality by design. AAPS J. 2014 Jul;16(4):771-83
- FDA, Guidance for Industry, Q2(R2) Validation of Analytical Procedures, March 2024, ICH-Quality Revision 2. https://www.fda.gov/media/161201/download
- 21 CFR Part 600 – Biological Products: General. https://www.ecfr.gov/current/title-21/chapter-I/subchapter-F/part-600
- 21 CFR Part 610 – General Biological Products Standards. https://www.ecfr.gov/current/title-21/chapter-I/subchapter-F/part-610
- David Keire, The Future is Now: ICH Q14: Analytical Procedure Development ICH Q2(R2): Validation of Analytical Procedures, May 24, 2022, CASSS AT Europe.
- Kunzelmann M, Wittmann A, Nold V, Presser B, Schreiber J, Gehrig T, Sadlers S, Scholz R, Solzin J, Berger A, Eppler K. Functional design of experiment for potency assay optimization and in-silico simulation. J Pharm Biomed Anal. 2023 Sep 20; 234:115584
- Gurba-BryĆkiewicz L, Maruszak W, Smuga DA, Dubiel K, Wieczorek M. Quality by Design (QbD) and Design of Experiments (DOE) as a Strategy for Tuning Lipid Nanoparticle Formulations for RNA Delivery. Biomedicines. 2023; 11(10):2752. https://doi.org/10.3390/biomedicines11102752
About The Authors:
Laura I. Salazar-Fontana, Ph.D., is a regulatory affairs professional with over 15 years of experience in the fields of quality, analytical method development/validation, translational sciences (biomarkers), and immunogenicity, accrued as a FDA CDER quality reviewer and successively in multiple leadership roles both in small and large pharmaceutical companies, including Genzyme, Sanofi, and Fresenius Kabi SwissBioSim. Her career spans all stages of drug development with hands-on expertise in the implementation of scientific- and risk-based approaches to the life cycle of biotherapeutics and recombinant peptides. She has co-authored multiple peer-reviewed publications in the field of immunogenicity and RA strategy. She is currently an expert consultant within NDA Partners, a Propharma Group company, founder of LAIZ Reg Science, and cofounder of the Immunogenicity Integrated consulting platform. She is a member of the Immune Responses Therapy committee in the American Society of Gene & Cell Therapy (ASGCT) and a special lecturer at the European Center of Pharmaceutical Medicine (ECPM), Faculty of Medicine at the University of Basel in Switzerland. She holds a Ph.D. in biochemistry and molecular biology, from the University Autónoma de Madrid in Spain.
Daniela Drago, Ph.D., RAC, is a partner at NDA Partners, a Propharma Group company. During her career, she held leadership roles in the pharma and biotech industries, consulting organizations, and academic institutions. In the industry, she held senior positions in global regulatory and medical affairs at companies ranging in size from startups to Fortune 500, including Roche, Biogen, and Bausch & Lomb. She was formerly an associate professor at George Washington University’s School of Medicine and presently has an adjunct appointment there in the Department of Pediatrics. Currently, she serves on the board of directors of MKB Biopharma and the Regulatory Affairs Professionals Society (RAPS). She is a fellow of RAPS and of The Organization for Professionals in Regulatory Affairs (FTOPRA). Daniela has received several awards, including the TOPRA Award for Regulatory Excellence and the RAPS Community Leadership Award. She received her Ph.D. in chemistry from the Swiss Federal Institute of Technology (ETH Zurich) in Switzerland.