Advanced, Cost-Saving Techniques Improving Cell Therapy Production
By Francisco Conesa BuendÃa
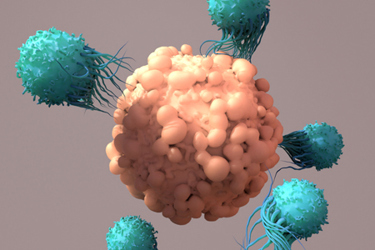
The manufacturing strategies described and implemented by cell therapy specialists have so far enabled the development of multiple CAR T-cell products and the demonstration of the potential of CAR T-cell therapies to overcome advanced malignancies. However, real-world experiences with CAR T-cell therapy following its FDA approval have also highlighted the limitations of conventional cell manufacturing processes.1 Translating these cutting-edge therapies from the lab to large-scale production remains a challenge due to the complexity of the manufacturing processes, which involve living cells, viral vectors, and stringent quality control requirements.2 In addition to the above, the low-throughput and intense resource demand limit patients' access to potentially life-saving therapies.1,2
The following describes different transformations or emerging techniques that could improve and monetize these therapies.
Accelerated Cell Manufacturing
In response to the challenges in CAR T-cell manufacturing, new strategies have been implemented to significantly shorten the manufacturing duration, thereby lowering production costs, reducing the likelihood of failures, and minimizing vein-to-vein time.3 These next-generation manufacturing processes can produce final formulations in as little as 24 hours, potentially expediting patient access to therapy.3 Frequently, these processes lead to saving time but also a substantial reduction in the ex vivo expansion period, which typically limits the number of cells produced. However, this decrease in ex vivo cell divisions may lead to a less differentiated T cell population, which could possess greater long-term proliferative capacity post-infusion. Early results suggest that products harvested at these earlier time points display a therapeutically advantageous phenotype and function.3
A theoretical concern regarding the accelerated manufacturing process is that CAR T cells cryopreserved shortly after ex vivo activation might show signs of overstimulation.3,4 Additionally, in the context of hematological malignancies, products from these shortened manufacturing processes may have a higher likelihood of containing residual tumor cells that would usually be eliminated during a longer ex vivo culture optimized for T cell growth. Nevertheless, existing clinical data indicate that the safety profile of CAR T-cell products generated through these expedited protocols remains clinically manageable.4
It is also crucial to recognize that regulatory requirements for product release testing still apply to those manufactured through accelerated processes, and the time needed for such testing must be considered when estimating vein-to-vein time.
Process Automation
One way to enhance commercial CAR T-cell production is through automation of the manufacturing process. This can lead to lower production costs, reduce the risk of human error and contamination, and loosen the need for highly skilled personnel.
Such automation supports the feasibility of on-site manufacturing, which can provide fresh non-cryopreserved products for patients.5 Notably, patients receiving fresh CAR T-cell products have shown significantly higher complete response rates (80% for fresh vs. 29% for cryopreserved), along with better CAR T-cell expansion and persistence compared to those treated with cryopreserved cells.6
While improvements in cryopreservation techniques could narrow this efficacy gap, these findings emphasize the potential benefits of on-site manufacturing.5 However, administering fresh products presents logistical challenges, requiring precise coordination between cell manufacturing and patient preparation for infusion. Additionally, the uniformity of automated systems can create difficulties when adjusting optimal process parameters for individual patients, as expansion rates may vary significantly, leading to differing medium requirements for cell culture. To achieve the best outcomes, instruments must be capable of monitoring critical parameters and adjusting as needed.
In Vivo Cell Manufacturing
Looking ahead, next-generation manufacturing processes may completely eliminate ex vivo cell manipulation and expansion and instead produce therapeutic cell populations in vivo.7 Several preclinical studies have demonstrated the ability to transduce T cells in vivo using lentiviruses or adeno-associated viruses.7 Furthermore, lipid nanoparticles (LNPs) and polymeric nanocarriers have been shown to deliver nucleic acid payloads to T cells in vivo.8 While absolute specificity in targeting T cells may not be essential, there could be benefits to concurrently producing CAR T cells, CAR natural killer cells, and CAR macrophages. It is crucial, however, to ensure that the transgene is not introduced into malignant cells, as this could lead to CAR proteins obscuring the target antigen and preventing CAR-expressing effector cells from recognizing the tumor.7,8 Additionally, off-target gene delivery raises the risk of genotoxicity associated with viral vectors capable of stable transgene integration.9 Therefore, it will be important to specifically direct the transgene delivery vehicle to T cells and to create strategies that enable efficient transgene integration without requiring external activation of T cells, thus producing an effective dose of CAR T cells with lasting antitumor effects.9 Despite the challenges ahead, bypassing ex vivo cell manufacturing could greatly reduce costs and improve access to CAR T-cell therapy for patients who require this treatment.
Digital And AI Integration
The integration of artificial intelligence (AI) and digital technologies could significantly enhance process development and optimization. Digital systems allow real-time monitoring and predictive analytics, ensuring that each batch of CAR T cells meets stringent quality standards.10 AI models can predict optimal culture conditions and outcomes based on data from previous runs, enabling faster optimization and reducing the need for costly trial-and-error experiments.10,11
Outlook And Future Perspectives
In conclusion, CAR T-cell therapy has rapidly transformed into a pivotal treatment for hematological malignancies, with its potential expanding into solid tumors and autoimmune conditions through ongoing clinical trials. The advancements in manufacturing processes have enabled the production of high-quality CAR T-cell products efficiently and reliably, ensuring that patients receive timely and safe treatments.
This evolution is underpinned by the accumulation of clinical knowledge, real-world data, and significant progress in automated manufacturing technologies, all of which contribute to refining the processes for autologous CAR T-cell production. Moreover, the dynamic field of cellular therapy is not limited to CAR T cells alone. The exploration of diverse immune-effective cells and the use of T cells from native populations present new avenues for research and application. These developments highlight a rich landscape of scientific innovation, offering the potential to address various malignancies and immune-related disorders more effectively. However, they also pose practical engineering challenges that must be addressed to ensure successful implementation.
Looking forward, the integration of advanced manufacturing techniques and a deeper understanding of T-cell biology will be crucial in overcoming these challenges. As the field continues to evolve, fostering collaboration between researchers, clinicians, and engineers will be essential to drive progress and enhance the efficacy and accessibility of CAR T-cell therapies. Ultimately, these efforts hold the promise of improving patient outcomes and expanding the frontiers of cancer treatment and immunotherapy, paving the way for a new era in personalized medicine.
References:
- Heathman TR, Rafiq QA, Chan AK, et al. 2015. "The Translation of Cell-Based Therapies: Clinical Landscape and Manufacturing Challenges." Regenerative Medicine. 10(1): 49–64. DOI: 10.2217/rme.14.73.
- Levine, B.L., Miskin J., Wonnacott K., and Keir C. 2016. Global manufacturing of CAR T cell therapy. Mol. Ther. Methods Clin. Dev. 4:92–101. DOI: 10.1016/j.omtm.2016.12.006.
- Yang, J., He J., Zhang X., Li J., Wang Z., Zhang Y., Qiu L., Wu Q., Sun Z., Ye X., et al. 2022. Next-day manufacture of a novel anti-CD19 CAR-T therapy for B-cell acute lymphoblastic leukemia: First-in-human clinical study. Blood Cancer J. 12:104. DOI: 10.1038/s41408-022-00694-6.
- Ghassemi, S., Durgin J.S., Nunez-Cruz S., Patel J., Leferovich J., Pinzone M., Shen F., Cummins K.D., Plesa G., Cantu V.A., et al. 2022. Rapid manufacturing of non-activated potent CAR T cells. Nat. Biomed. Eng. 6:118–128. DOI: 10.1038/s41551-021-00842-6.
- Elsallab, M., and Maus M.V. 2023. Expanding access to CAR T cell therapies through local manufacturing. Nat. Biotechnol. 41:1698–1708. DOI: 10.1038/s41587-023-01981-8.
- Shah, N.N., Johnson B.D., Schneider D., Zhu F., Szabo A., Keever-Taylor C.A., Krueger W., Worden A.A., Kadan M.J., Yim S., et al. 2020. Bispecific anti-CD20, anti-CD19 CAR T cells for relapsed B cell malignancies: A phase 1 dose escalation and expansion trial. Nat. Med. 26:1569–1575. DOI: 10.1038/s41591-020-1081-3.
- Michels, A., Ho N., and Buchholz C.J. 2022. Precision medicine: In vivo CAR therapy as a showcase for receptor-targeted vector platforms. Mol. Ther. 30:2401–2415. DOI: 10.1016/j.ymthe.2022.05.018.
- Smith, T.T., Stephan S.B., Moffett H.F., McKnight L.E., Ji W., Reiman D., Bonagofski E., Wohlfahrt M.E., Pillai S.P.S., and Stephan M.T. 2017. In situ programming of leukaemia-specific T cells using synthetic DNA nanocarriers. Nat. Nanotechnol. 12:813–820. DOI: 10.1038/nnano.2017.57.
- Michels, K.R., Sheih A., Hernandez S.A., Brandes A.H., Parrilla D., Irwin B., Perez A.M., Ting H.A., Nicolai C.J., Gervascio T., et al. 2023. Preclinical proof of concept for VivoVec, a lentiviral-based platform for in vivo CAR T-cell engineering. J. Immunother. Cancer. 11:e006292. DOI: 10.1136/jitc-2022-006292.
- Bäckel N, Hort S, Kis T, Nettleton DF, Egan JR, Jacobs JJL, Grunert D, Schmitt RH.2023 Elaborating the potential of Artificial Intelligence in automated CAR T cell manufacturing. Front Mol Med. 3:1250508. DOI: 10.3389/fmmed.2023.1250508.
- Aguilar-Gallardo, C., Bonora-Centelles A. 2024. "Integrating Artificial Intelligence for Academic Advanced Therapy Medicinal Products: Challenges and Opportunities" Applied Sciences 14, no. 3: 1303. DOI: 10.3390/app14031303.
About The Author:
Francisco Conesa Buendía, Ph.D., has been working in the field of advanced therapies since 2021 in Spain and the U.S. He has focused his research and work on the development of ATMPs based on stem cells and chondrocytes. Currently, he is a cell manufacturing assistant working on manufacturing and process optimization of cell and gene therapies based on CAR-T and T cells at Memorial Sloan Kettering Cancer Center in New York City. Connect with him on LinkedIn.