ADCs Are The Future Of Precision Chemotherapeutics
By Hyun Yong Cho and Byeong Sung Lee, Pinotbio, Inc.
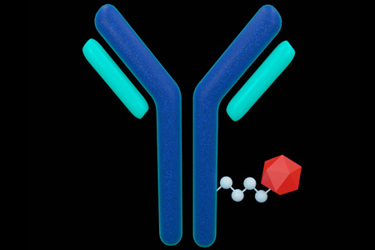
Chemotherapy has always been a delicate balance between maximizing tumor cell death and minimizing off-target toxicity. Increasing adverse side effects from small molecule chemotherapeutics can prevent patient compliance, requiring new precision medicine solutions to bring hope to this field.
Antibody-drug conjugates (ADCs) allow potent chemotherapeutics to be targeted to their intended site of action. These tripartite structures, consisting of a targeting antibody and drug payload connected via a linker, render the drug inert as it circulates the body until it is released and activated in the target cell. The targeting specificity and local release of cytotoxic chemotherapeutics within the tumor micro-environment provide increased anti-tumor efficacy and a decreased systemic toxicity. As a result, ADCs offer a wider therapeutic window compared to traditional systemic chemotherapy.1
Pharmaceutical companies are embracing the concept of ADCs, with 12 ADCs approved by the FDA,2 and advances in the development of targeted delivery vehicles over standard antibodies are improving the performance of these drugs and expanding their potential for the treatment of new cancers.
How ADCs Provide Targeted Treatments
The antibody component of the ADC acts as a precision nanocarrier for the chemotherapeutic payload. Selection of the best target tumor antigen on the cell surface can be a key determinant of ADC activity. Optimal ADC antigens are highly expressed on the tumor cell surface with restricted or no expression on normal (healthy) cells.
Highly selective antibodies for a tumor-associated antigen and minimal immunogenicity are the essential characteristics of ADC antibodies. This functionality prevents cross-reactivity to other antigens, preventing the ADC from being distributed to other tissues and target cells before reaching the intended site of action and causing off-target toxicity.
Upon target recognition and binding of the antibody on the tumor cell surface, rapid internalization of the ADC is important for effective payload delivery. Rapid internalization increases both the ADC’s effectiveness and safety, as this limits the potential for off-target release.3 To promote rapid internalization, it is important that the correct antigen is targeted by the antibody portion of the ADC and that the antibody (or antibody alternative) efficiently binds to the targeted antigen (Figure 1 below; click on image to enlarge).
Figure 1: ADCs utilize multiple mechanisms of action: (i) target recognition and cell internalization driven by the antibody, (ii) linker chemistry that allows payload deposition intracellularly and within the tumor microenvironment, and (iii) tailored payloads that ensure the drug remains within the targeted cancer cells and prevent drug resistance. Click on image to enlarge.
ADC internalization optimally occurs via the endocytic pathway. Once the ADC binds to its respective tumor antigen, it is internalized into endosomes that mature and fuse with lysosomes.4,5 Within the lysosome compartment, the drug is released from the ADC through cleavage of its linker by specific proteases, such as cathepsin B, or by degradation of the ADC. Newer ADCs such as PINOT-ADC PBX-001 use multiple payload release strategies, combining pH-sensitive cleavable linkers that allow payload release within the tumor microenvironment or following internalization within the endosomal compartment.6 This is beneficial because internalization of the ADC via clathrin-coated pit-mediated receptor internalization into the caveolar pathway may result in it being trafficked to the Golgi or endoplasmic reticulum.7 These organelles lack the required proteolytic (or low pH) function found in lysosomal compartments, potentially impeding the release of the chemotherapeutic portion and the effectiveness of the ADC.8
Engineering Collateral Damage With The Bystander Effect
Following ADC processing within the lysosomal compartment, the free drug can traverse the lysosomal membrane into the cytosol, bind its target, and trigger cell death. Studies have also shown that free drug is able to cross the plasma membrane and access the extracellular space targeting neighboring cells in a process known as the bystander effect.9-11 Emerging evidence suggests that internalization may not even be essential to cause the bystander effect if degradation of the ADC occurs in the extracellular space.12
The extent to which an ADC mediates bystander killing depends on several factors: the degree of ADC internalization upon binding the tumor antigen, the presence of a non-cleavable or cleavable linker, and the hydrophobicity of the attached cytotoxic payload. For example, Roche’s Kadcyla, ado-trastuzumab emtansine, used for the treatment of HER2-expressing metastatic breast cancer,13 delivers DM1 (a positively charged drug payload) attached via a non-cleavable thioether linker. As such, this ADC must be internalized to allow antibody degradation, rather than degradation of the linker, to release the drug. As a result of the positive charge of the drug payload, the cleaved product is unable to penetrate the cell membrane to enter surrounding cells,14 reducing the bystander effect.
While reduced bystander-induced death may be preferable to prevent drug resistance, this feature may also be desired to increase cell death within the center of solid tumors. PINOT-ADC uses a pH-sensitive linker that can be cleaved within the tumor microenvironment prior to cell internalization or within the endosome following internalization (Fig. 1). Combining this feature with the hydrophobicity of the novel camptothecin payload FL-118, which prevents the drug being pumped out of the cell via efflux pumps, allows increased cell-specific killing and utilizes the bystander effect within the tumor microenvironment.6,15 These factors can be engineered into the ADC as required and are increasingly taken into account in the development of ADCs, as they imply that targeting a subset of antigen-expressing cells within the tumor can still effectively deliver the drug to a larger tumor population.
Delivering New Solutions For ADCs
Antibodies have not evolved to deliver payloads and have intrinsic limitations in this capacity. Emerging trends in ADC development show an increasing number of novel format affinity ligands being employed in place of antibodies for antigen-targeting purposes. These novel format ligands include protein scaffolds, peptide scaffolds, and oligonucleotides as aptamers. One example of this is a collaboration between PinotBio and Aptamer Group to develop Optimer-drug conjugates (ODCs), replacing the targeting antibody with an aptamer-based oligonucleotide affinity ligand. Alternative affinity ligands are selected from in vitro libraries and engineered to deliver performance improvements as targeted delivery vehicles. Also, due to development being purely in vitro, they can give access to new target antigens, including toxic or non-immunogenic target antigens, enabling improved precision chemotherapeutics.
Moving To Miniature
Many novel format ADCs utilize affinity ligands much smaller than the standard 150 kDa antibody. Both aptamers and nanobodies have shown improved extravasation and improved tissue penetration, with regard to both depth and speed of tissue penetration, into perfused solid tumors due to their smaller size.16,17 Improved penetration of solid tumors increases payload delivery to the internal tumor microenvironment for more effective ADCs.18,19
Tailored Affinity And Half-life
In vitro discovery and development of novel format delivery vehicles offers the potential to tune critical characteristics, such as half-life and target affinity.
While many antibodies used in ADCs are selected for their long half-life, this can lead to uncontrolled payload release and toxicity in non-target tissues. Shorter retention times available with novel format delivery vehicles have been shown to prevent this. For example, Bicycle Therapeutic’s BT5528, a ~4 kDa bicyclic peptide (Bicycle) targeted to EphA2 with an auristatin-derivative payload, showed improved safety profiles compared to the ADC MEDI-457 that comprises an EphA2-targeting antibody with an auristatin payload.20 MEDI-457 was terminated in Phase 1 trials due to treatment-related bleeding and coagulation events.21 The ability to tune ADC half-life so it’s dependent upon the payload and disease characteristics being addressed will be important for the success of each precision chemotherapeutic in development.
While high-affinity binding may be preferable for targeting circulating tumor cells in the treatment of hematological cancers, when targeting solid tumors, ligands that exhibit lower target affinity have been shown to improve tumor penetration.22, 23 Being able to tune the affinity of the delivery vehicle, along with smaller molecular size of novel format ADCs, may offer methods to marry the benefits of improved penetration into solid tumors with increased cell death via toxic payload delivery and greater bystander effects.
Simpler DAR
Optimization of the drug-antibody ratio (DAR) has resulted in improved in vivo efficacy and safety. Antibody-payload conjugation has historically used lysine residues in the antibody. With more than 80 residues in each antibody, this conjugation method is inconsistent and requires extensive post-manufacturing work to ensure standardization of the final product. Site-specific conjugation chemistries, targeting cysteine residues for stable thiol linkages, allow increased consistency in DAR optimization. However, targeting cysteine residues within antibodies can be challenging as many of the natural cysteines in these molecules are essential for stabilizing the antibody structure. The process of reverse-engineering new cysteine residues into an antibody requires extensive development and can disrupt native disulfide bridges. A more straightforward emerging solution is the use of novel format ligands that can be engineered with additional cysteine residues at specific positions for site-specific conjugation. Aptamers and many of the alternative protein scaffold ligands do not contain cysteine residues, allowing the easy integration of these residues for targeted site-specific conjugation to aid straightforward and consistent DAR in the final product.
Preventing Immunogenicity
For many protein scaffold-based novel format ADCs currently entering development pipelines as targeting moieties, a key consideration is the immunogenicity of the scaffold protein itself. Though these protein ligands offer significant advantages over antibodies, the potential for each ligand to generate adaptive immune responses must be assessed.
As an alternative, aptamers are composed of modified oligonucleotides, which typically show minimally or non-immunogenic profiles. Along with the ability to optimize half-life, affinity, stability, site-specific conjugation, and their low molecular weight, this gives aptamers an optimal profile for the next generation of ADCs.24
Identifying New Biomarkers
Due to the nature of antibody development, not all targets are compatible with the generation of fit-for-purpose antibodies for use in ADCs. Employing novel format alternatives25 can expand this potential target range to create a wider pool of tools upon which the industry can draw to deliver best-in-class molecules. Furthermore, as we progress into the development of ADCs for less well-defined cancers, we can investigate biomarker-free affinity ligand discovery. Biomarker-free discovery is performed without a priori knowledge of the specific oncological biomarker, with screening taking place against cancerous and healthy cells to discriminate Optimer sequences that bind only to the cancer cells of interest.26 Biomarker ID can then be carried out retrospectively, using the identified Optimer to isolate the target ligand, using methods such as mass spectrometry. This opens the door to identifying new cancer biomarkers for improved targeting of ADCs and new diseases that may be pursued using the next generation of ADCs.
ADCs have evolved through multiple generations to improve cancer treatment and patient outcomes. As we look to the future of precision chemotherapy, leaders in this space are examining which novel technologies and approaches will enable further improvements in the therapeutic window and help position ADCs as frontline treatments. Established antibody biologics have proven drug profiles that can be readily adapted to develop ADCs for specific targets and diseases. However, the use of novel format delivery vehicles is showing much promise for fully optimized delivery conjugates that can be tailored to the biomarker, payload, and disease.
References
- Teicher BA, Chari RV. Antibody conjugate therapeutics: challenges and potential. Clin Cancer Res. 2011;17(20):6389–97.
- Webster P. A golden era of cancer clinical trials. Nat Med. 2022;28:602-605.
- Khandelwal A, et al. Antibody-drug conjugate development. In: Phillips GL, editor. Antibody-drug conjugates and immunotoxins. New York: Human Press; 2013. p. 23–38.
- Peters C, Brown S. Antibody-drug conjugates as novel anti-cancer chemotherapeutics. Biosci Rep. 2015;35(4).
- Parslow AC, et al. Antibody-drug conjugates for cancer therapy. Biomedicine. 2016;4(3):1-17.
- PinotBio. Available at: https://www.pinotbio.com/en/m34.php#main
- Le PU, Nabi IR. Distinct caveolae-mediated endocytic pathways target the Golgi apparatus and the endoplasmic reticulum. J Cell Sc 2003;116(Pt 6):1059–1071.
- Katz J, et al. Brentuximab vedotin (SGN-35). Clin Cancer Res 2011;17(20):6428–6436.
- Kovtun YV, et al. Antibody-drug conjugates designed to eradicate tumors with homogeneous and heterogeneous expression of the target antigen. Cancer Res. 2006;66(6):3214–21.
- Kovtun YV, Goldmacher VS. Cell killing by antibody-drug conjugates. Cancer Lett. 2007;255(2):232–40.
- Sammet B, et al. Antibody-drug conjugates in tumor therapy. Pharm Pat Anal. 2012;1(1):65–73.
- Staudacher AH, Brown MP. Antibody drug conjugates and bystander killing: is antigen-dependent internalisation required? British Journal of Cancer. 2017;117:1736-1742.
- Verma S, et al. Blackwell K Trastuzumab emtansine for HER2-positive advanced breast cancer. N Engl J Med. 2012;367(19): 1783–1791.
- Erickson HK, et al. Antibody-maytansinoid conjugates are activated in targeted cancer cells by lysosomal degradation and linker-dependent intracellular processing. Cancer Res. 2006;66(8): 4426–4433.
- Staudacher AH, Brown MP. Antibody drug conjugates and bystander killing: is antigen-dependent internalisation required? Br J Cancer. 2017; 117(12):1736-1742.
- Debie P et al. Size and affinity kinetics of nanobodies influence targeting and penetration of solid tumours. J Controlled Release. 2020;317:34-42.
- Xiang D et al. Superior Performance of Aptamer in Tumor Penetration over Antibody: Implication of Aptamer-Based Theranostics in Solid Tumors. Theranostics 2015;5(10):1083-1097.
- Thurber GM, Dane Wittrup K. A mechanistic compartmental model for total antibody uptake in tumors. J Theor Biol 2012;314:57–68.
- Li Z, et al. Influence of molecular size on tissue distribution of antibody fragments. mAbs. 2016; 8:113–9.
- Bennett G. MMAE Delivery Using the Bicycle Toxin Conjugate BT5528. Mol Can Therapeutics 2020; 19(7):1385–1394.
- Annunziata CM et al. Phase 1, open-label study of MEDI-547 in patients with relapsed or refractory solid tumors. Invest New Drugs. 2013;31(7):77-84.
- Rudnick SI et al. Influence of Affinity and Antigen Internalization on the Uptake and Penetration of Anti-HER2 Antibodies in Solid Tumors. Cancer Res. 2011;71(6):2250-2259.
- Evand R, Thurber GM. Design of high avidity and low affinity antibodies for in situ control of antibody drug conjugate targeting. Sci Reports 2022;12:7677.
- Aptamer Group. Available at: https://aptamergroup.com/applications/therapeutics/
- Optimer targets. Available at: https://aptamergroup.com/optimer-binders/optimer-targets/
- Scientist.com. Cutting Edge Conversations: Discovering new innovations in Oncology. 2022. Available at: https://insidescientific.com/webinar/cutting-edge-conversations-discovering-new-innovations-in-oncology/
About The Authors:
Hyun Yong Cho is chief scientific officer at Pinotbio, Inc. He is in charge of development of next-generation antibody-drug conjugate (ADC) candidates and platforms. He obtained his Ph.D. in biomolecular engineering at the Korea Advanced Institute of Science and Technology. He built up his career throughout all the stages of chemical product commercialization, including fundamental research on organic chemistry-based semiconductor materials, process design, and development at Samsung Cheil Industries (Electronic Materials Division, Samsung SDI, at present) and Heraeus, a German fine chemicals company.
Byeong Sung Lee leads the drug discovery team at Pinotbio, Inc. He obtained his Ph.D. in biotechnology at Ajou University, Korea, and then studied bioconjugation at the Molecular Science and Technology Research Center, Ajou University, as a researcher. His research focus is protein engineering and antibody-drug conjugation.