A "Particular" Problem For Cell Therapy Products, Part 2: Cell Clumps And Other Inherent Particles
By Drew N. Kelner and Andreas M. Beckhaus, ProDeMaCon LLC Consulting
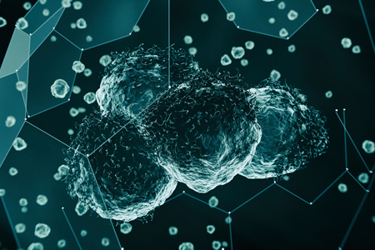
In part 1 of our article series about “particular problems” in cell therapy products, we focused on extrinsic and intrinsic particles and provided recommendations on how a cell therapy manufacturer can create a risk management strategy. Part 2 focuses on the third type of particle class,1 inherent particles. These particles are expected from the drug product itself and may — within limits — represent a generally accepted characteristic of the product (e.g., protein aggregates). In the case of cell therapy products, a special type of inherent particle, i.e., cell clumps, has so far received limited attention.
Cell therapy manufacturers need to understand the potential impact of cell clumps that may be present prior to administration of their final drug product (FDP). In the absence of significant regulatory guidance in this newly emerging form of therapeutic, the manufacturer needs to establish a risk management strategy for control of particulates.
Background
The typical CAR-T product labeling includes statements about cell clumps. For example, in the case of Kymriah,2 the label states: “Inspect the contents of the thawed infusion bag for any visible cell clumps. If visible cell clumps remain, gently mix the contents of the bag. Small clumps of cellular material should disperse with gentle manual mixing. Do not infuse … if clumps are not dispersed ...”
To our knowledge, Carvykti is the only licensed cell therapy product using an IV administration set with an in-line non-leukocyte depleting filter for bedside patient administration. This filter has the potential to reduce the amount of (at least) larger cell clumps that would otherwise be administered.
The potential safety risks from administered cell clumps can be divided into two categories: physiological risk and immunological risk.
Physiological Safety Assessment
Particles administered intravenously can impede and even block blood flow. Depending on their size and origin, particulate matter can become trapped in the pulmonary capillaries within the lungs, the diameter of which is approximately 12–15 μm. Particle entrapment can result in chronic inflammation that can, over time, lead to vessel occlusion, thrombo-embolism, and other forms of cardiovascular damage.
While significant data has accumulated regarding particulates in protein drugs, the cell therapy field is newer, and by its nature, the administration of cellular materials introduces additional particulate risks not found in (bio)pharmaceutical drug products: cell debris and cell clumps. Physiologically, the most significant concern is the potential of cellular aggregates to block small vessels. The diameter of a single activated T cell is approximately the same as that of the capillary bed, whereas a clump of T cells can be substantially larger, perhaps as large as 1,000 µm in diameter.3 It is therefore feasible that T cell clumps may cause physiological damage.
When arrested in capillary beds, red blood cells easily undergo structural deformation and eventually get through the capillary bed after multiple passes by the blood through the lungs. T cells, and even clumps of T cells, are also capable of deformation. However, due to significant structural and functional differences between erythrocytes and T lymphocytes, it is highly likely that T cell clumps do not readily transit through restricted capillary beds like red blood cells (RBCs), because:
- RBCs have no nucleus or organelles. They are essentially plasma membranes full of hemoglobin and a few other proteins, built for easy deformation and passage through small vessels. Lymphocytes have large nuclei and a full complement of cytoplasmic organelles and therefore they are less compressible than RBCs.
- Erythrocytes tend to avoid contact with the endothelial cells lining the vessels. The complex fluid dynamics of blood results in a concentration of erythrocytes across the cross-section of blood vessels; the highest concentration of RBCs remain in the middle of the vessels and the lowest at the walls, creating an RBC-free zone at the periphery.4 Lymphocytes, on the other hand, tend to circulate in proximity to the vessel walls5 and adhere to endothelial cells as part of their immunological function during the inflammatory response, in which proteins called integrins on the T cell surface bind to the extracellular matrix of cells in areas of inflammatory damage.
Because T cell clumps are unlikely to easily pass through capillary beds, they pose a potential thromboembolism risk. A study with mesenchymal stem cells (in the 18-30 µm range) showed that the cells were easily trapped in the lungs, with the larger cells showing a greater tendency for lung arrest than the smaller cells.6
Materials that are sometimes embedded in cell clumps present an additional risk. The cell clumps can contain potentially toxic substances, such as metals and process-derived leachates. Such materials, when liberated from the aggregated cells, can be taken up into local cells by endocytosis. Internalization of these substances can disrupt multiple cellular functions, including respiration, metabolism, and energy utilization. The cellular stress induced by such disruptions can lead to apoptotic signaling with subsequent death of the affected cell.
Immunological Safety Assessment
The human immune response is remarkably sensitive, such that all drug products manufactured in living cells carry immunological risks. The art and science of immunogenicity prediction for protein-based drugs have matured significantly over the past few decades. These developments include useful algorithms for predicting immunogenicity. The cell surface is studded not with one but many different proteins, thereby creating a far more complex immunological issue for which we lack sufficient tools for predictive analysis. It is important to note that our current understanding of the physiological impact of particulates in biological drugs is based on our vast experience with protein drugs. Due to our limited experience with cell and gene therapies, the particulate risk assessment for these new therapeutic modalities requires additional scrutiny beyond the current state of knowledge. As an example, the immune response is highly sensitive to molecular patterns. Herein we find the reason why some of the recombinant viral vaccines are multivalent constructs of one or more viral proteins. Pattern recognition is an important mechanism driving innate responses by macrophages, neutrophils, and natural killer cells, which recognize antigenic arrays on pathogens such as yeast, bacteria, and other pathogens. Cellular debris and cell clumps contain complex molecular patterns not found in the case of protein drug aggregates.
At the heart of the adaptive immune response elicited by B and T cells is the ability to discriminate between “self” and “non-self” antigens. While engineered T cells might lack the TCR and other surface antigens (like CD52), many other antigens are found both inside and on the surface of the cell. While autologous T cells contain “self-antigens” (with the exception of the scFv domain of the CAR construct), allogeneic T cells contain additional “non-self” antigens. While aggregation of biological materials can be immunogenic in both instances, the likelihood that a cell clump containing “non-self” antigens in an allogeneic FDP might elicit an immune response (including an anti-product immune response that reduces therapeutic efficacy) is greater than for a self-antigen-containing aggregate in an autologous cell clump. Such a response can enhance inflammation resulting from the presence of entrapped cells in small vessels, stimulating inappropriate cytokine release that can increase the severity and/or duration of the well-known cytokine release syndrome (CRS) in immunotherapy patients.
Recommended Risk Mitigation Steps And Studies
FDP control measures for inherent visible particles should be implemented by manufacturers of cell therapy products. USP<1046>7 indirectly implies that certain inherent particulate matter may be expected in cell therapy products and states that cell clumping may be present as long as it is not “excessive.” Vials with visible cell clumps should be compared to pictures in a defect library that represent pure cell clumps of certain sizes during the drug product vial inspection process. Vials with visible cell clumps may be acceptable, provided an in-line filter is used for administration (see above). Different filter mesh types and sizes should be studied with FDP vials containing cell clumps to determine if the filtration is effective (e.g., with cell size distribution analysis) and/or if the administration line is susceptible to clogging by cell clumps.
This strategy should also be supported by studies with final diluted product for administration. To determine if certain cell clumps visible in the administration will dissolve by mixing (as indicated in other products’ instructions), a study should be conducted with cryopreserved product vials. This study should include vials with different levels of cell clumps as well as vials without cell clumps. All vials should be prepared for administration as per instructions for use. Syringes containing cell clumps should be “gently mixed” and assessed to determine what kinds of cell clumps may or may not dissolve.
Conclusions
Cell therapy manufacturers need to develop a sound particulate control strategy as soon as they move into clinical trials, covering all three classes of particles, including cell clumps. As noted, while clumps and cellular debris from autologous CAR-T therapies are largely composed of self-antigens and thereby present lower immunological risk, both the surface and intracellular proteins in allogeneic cells are composed of non-self-antigens, all of which are subjected to scrutiny by the innate immune system and subsequently presented to host T cells. Therefore, the immunological risk from allogeneic cells is likely greater than that of autologous cells. We cannot ascertain the degree of risk inherent in “non-self” vs. “self” T cell therapies due to limited experience and a lack of clinical safety data. It is therefore incumbent upon the manufacturer to implement a rigorous risk assessment for particulate matter in both types of cell therapy products that considers the biological differences between therapeutic agents derived from autologous and allogeneic T cells.
References
- Clarke D, Stanton J, Powers D, Karnieli O, Nahum S, Abraham E, et al. Managing particulates in cell therapy: Guidance for best practice. Cytotherapy 2016;18:1063–76
- https://www.novartis.com/us-en/sites/novartis_us/files/kymriah.pdf
- Li M, Chin LY, Shukor S, Tamayo AG, Maus MV, and Parekkadan B. Effects of Intermittent T-Cell Cluster Disaggregation on Proliferative Capacity and Checkpoint Marker Expression. Autoimmunity. 2019 May; 52(3): 102–107. doi:10.1080/08916934.2019.1630064.
- Secomb TW. Hemodynamics, Compr Physiol. ; 6(2): 975–1003. doi:10.1002/cphy.c150038
- Fedosov DA and Gompper G. White blood cell margination in microcirculation. Soft Matter, 2014, 10, 2961 DOI: 10.1039/c3sm52860j
- Cui L, Kinnunen, K, Boltze J, Nystedt, J and Jolkkonen, J. Clumping and Viability of Bone Marrow Derived Mesenchymal Stromal Cells under Different Preparation Procedures: A Flow Cytometry-Based In Vitro Study. Stem Cells Int. 2016: 1764938
- USP<1046> Cellular and Tissue-Based Products
About The Authors:
Drew N. Kelner is president of Shenandoah Biotechnology Consulting, LLC and a principal consultant at ProDeMaCon LLC. In a 37-year career in the biotechnology industry, he has participated in the development of monoclonal antibodies and other biopharmaceutical products from both bacterial and mammalian cell sources as well as oncolytic viruses and CAR-T therapies. He retired from Amgen in 2015 from his position as executive director of global analytical sciences. Kelner is the author of Taming Cancer: 21st Century Biology and the Future of Cancer Medicine. With a background in protein biochemistry and molecular immunology, he received a B.S. in chemistry from Haverford College and a Ph.D. in biochemistry from Duke University.
Andreas M. Beckhaus is the president of ProDeMaCon LLC. He started his international career in the regulatory affairs department of Bayer Healthcare and in the following 17 years worked in several different functions (project management, life cycle management, portfolio management, and marketing) with increasing responsibility, focusing on biotech products and rare diseases. He further broadened his scope into medical devices at KCI Inc., a global wound-healing company. In 2014, Beckhaus started ProDeMaCon as an independent consulting business. Over the last several years, he worked with several clients in the CGT space (small and large pharmaceutical companies and CDMOs) with a focus on raw material qualification and particulate control strategies. He has a background in pharmacy and a Ph.D. in toxicology.