Utility System Design Considerations For Single-Use Bioprocessing
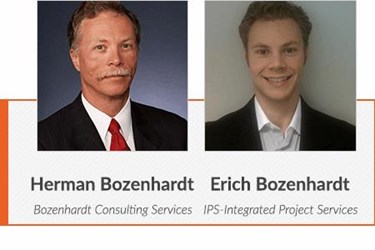
By Herman Bozenhardt, Bozenhardt Consulting Services, and Erich Bozenhardt, IPS-Integrated Project Services
Expansions and renovations to existing biological facilities, and construction of new facilities, provide a unique opportunity to rethink basic design strategies and use new technologies to build a better facility that will improve compliance. This article is the fifth in a six-part series on how single-use systems are changing the modern biotechnology facility and process design paradigm.
As discussed in previous articles in this series, newly designed biotech facilities are profoundly different from the legacy facilities we are all used to seeing, including those built as recently as five years ago. Facilities following a modern rapid-deployment/delayed-capital philosophy provide one of the most striking differences: the absence of traditional utility systems. This article will discuss the trends in utility system design, the rationale behind them, and a concept for the future.
An Overview of Utility Systems for Bioprocessing
Utility systems are capital-intensive, have long lead times, and are not value-additive, but they do provide critical support for the process and operations. The current industry emphasis on working capital management is driving the development of innovative ways to consolidate and eliminate many traditional utility systems. With the application of single-use systems (SUS), a number of factors have become evident in utility system design:
- The vast majority of utility demand in a traditional biotech facility comes from the water systems and plant steam / clean steam systems required for washing, cleaning, flushing, and sterilization. This alone can contribute up to 25 percent of the facility’s footprint and can have a profound impact on floor-to-floor spacing and the need for utility chases.
- Unless the process is a perfusion cell culture, the actual water demand for direct or continuous use is relatively small compared to the water for cleaning and sanitization.
- There is not a universal philosophy that works for every facility. However, if WFI is needed for compounding, a small contained RO/EDI (reverse osmosis / electrodeionization) on-demand system could be employed for limited use.
- Water for injection (WFI) can be bought in quantity and treated like all raw materials, thus eliminating substantial equipment, maintenance, validation, and testing. Water-, WFI-, and steam-related systems represent nearly half of the maintenance costs in traditional biopharmaceutical facilities.
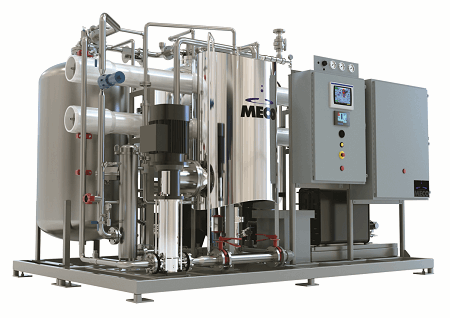
Image credit: MASTERpak water purification system, courtesy of MECO
- With increased product sensitivity to oxygen and more products with low potencies, nitrogen for product blanketing will continue to be necessary. In a facility with SUS, we are seeing the use of nitrogen pressure as a substitute for pumping liquid from one bag to another. With the need to assure inert blanketing, some products are being purged with argon, which is substantially heavier than air and greatly reduces diffusion (unlike nitrogen).
- Historically, process drain systems within any pharmaceutical facility were extensive and segregated by process (clean in place, sterilization in place, non-potent process), potent or liquid waste requiring post-treatment, and the sanitary system. New facilities may have limited drains for condensate and cleaning solution make-up, as the residual process liquids will be contained in a bag, sealed, and sent off for disposal. The only new requirement is to label and track the waste for the waste processor to which it will be sent.
- Clean steam is only necessary if an autoclave is required for on-demand sterilization/decontamination of a part or container. However, that application should be evaluated to determine if a disposable item can be used instead.
- Clean dry air (CDA, .22 micron filtered) is necessary for control valves.A single CDA grid can be installed to handle any air requirement, providing a clean source.
- A detailed analysis will be required to justify any utility system with an overwhelming emphasis on the single-use philosophy. In addition, the potential always exists to consolidate systems, such as the elimination of WFI, deionized (DI), and process potable water grids in favor of one WFI.
Specific Utility Considerations
With the limited use of clean steam and WFI in single-use facilities, combination systems (multiple-effect still with clean steam from the first effect, or a clean steam generator that feeds a condenser) are typical. A recent change in the European Pharmacopeia for WFI requirements now makes an RO/EDI system a viable option for small ambient WFI systems.
U.S. Pharmacopeia (USP) / Deionization (DI)
With the emergence of options for combination water delivery (as well as make or buy options), the old concept of a USP/DI system will ultimately disappear in favor of a cheaper (less capital-intensive) point-of-use WFI. The key is viewing the reduced water requirements as an opportunity to combine and integrate (maybe eliminate) into a minimalistic footprint.
Electrical Power
Although it is obvious that power grids will be extensive, it is important to note that most new facilities are incorporating some features of LEED (Leadership in Energy & Environmental Design), energy conservation, and self-generation to lower costs, garner tax incentives, and to be more responsible to the local environment.
Inert Gases
Clearly, there is a need to continue and potentially expand the role of inert gasses. The only real challenge here is correctly calculating the use and sizing of the storage vessel.
Clean in Place (CIP)
As we have discussed, CIP will generally disappear with the use of disposables; however, there may still be the need for parts washers. These can be outfitted with their own water generation systems, provided power and potable water are supplied.
Clean Steam
The need for clean steam becomes very limited in the design of a new biotech plant. The product manager and the designer should explore the options of product campaigning to acquire presterilized components before the batches. Use of clean steam for humidification can be replaced with DI water/plant steam exchangers.
One large biotech firm built a decontamination autoclave (dedicated clean steam generator) and padded the design by quadrupling the size for surge capacity. They realized after startup they would only need to use the unit once a month for 10 liters of lab waste and once a quarter for one 20 liter bag of process waste. The decontamination could have been done with a simple bottle of bleach. Capacity planning and practicality is more important than doing what the legacy facilities have done.
As an example of innovation from the aseptic world, the use of closed vial filling has eliminated the need for vial washers, sterilizing tunnels, and stopper handling. The footprint of the aseptic facility of the future will be substantially smaller and will resemble an automated packaging plant.
Chilled Water
A chilled water plant will always be needed to drive the HVAC and process cooling systems. However, in the world of SUS, the energy load is radically reduced without the traditional CIP/SIP operations.
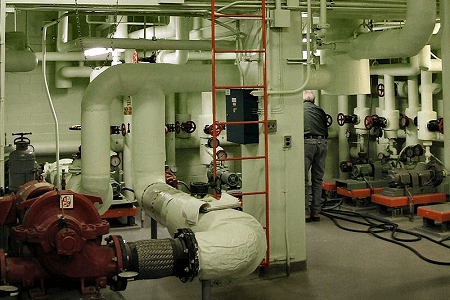
Image credit: Mechanical room.jpg by P199 - http://nyphotographic.com/. Licensed under CC BY 2.5 via Wikipedia.
Plant Steam
If local power costs are high and fuel costs are low, plant steam can provide a means to both meet facility heating demand and generate clean steam.
CDA (Clean Dry Air)
A common supply system with local filtration and pressure regulation can serve both instrumentation users and process users, allowing this critical utility to be driven by the automation needs rather than process needs. The one important issue is to recognize the need for CDA if using air-driven agitators or pumps. Air-driven equipment is required in Class I, Division 1 or 2 areas where solvents or explosive dusts are present.
Drain Systems
Drains are the forgotten utility. However, they can create runaway costs in the absence of critical thinking and consolidation (e.g., in-situ neutralization and decontamination for on-the-floor activities).
Process Thermal Control Units (TCUs) / Hot Water Systems
Hot water grids have slowly disappeared over the last several years; however, elevated temperature is still needed for process reactors. The easiest solution is wheeled (mobile) electrically powered TCUs, which provides hot water from a potable water or chilled water source, have onboard temperature controls, and can interface to programmable logic controllers (PLCs) or distributed control systems (DCSs) for remote control. These are cost-effective, multipurpose systems that can be cleaned and moved around a production floor or mechanical chase for plug-and-play applications.
Sterilizing Gas Systems
Although mostly relegated to the aseptic fill end of our business, biotech firms are being asked to provide more handling, bulk filling and small batch sizing filling. The primary gases / sterilizing agents used are VHP (vaporized hydrogen peroxide), iHP (ionized hydrogen peroxide), chlorine dioxide, and nitrogen dioxide. Although VHP has been the industry’s dominant agent, the dawn of ultra-low potency products and highly sensitive biologicals has challenged this dominance. VHP forces firms and designers to choose materials, hoses, and tubing that do not attract and retain HP; build in ultra-long degassing cycles in isolators; and struggle with temperature and relative humidity (RH) to promote the kill cycle, eliminate condensation, and ensure complete aeration.
Today, designers need to ask the critical question of sterilant compatibility with products first. While iHP provides a better cycle control, the use of HP forces us to consider ClO2, which is very effective but could face similar compatibility issues. We believe consideration long-term favors nitrogen dioxide, which appears to degrade faster and have less material impact. In all our choices, we must build-in in situ gas generators, provide utilities and delivery infrastructure for them, and analyze all the risks and safety concerns each bears.
Conclusion
Rapid-deployment/delayed-capital single-use facilities can realize a major reduction and elimination of certain utilities. The designer must first engage the user organization to understand the plant capacities and production objectives, in order to generate a realistic utility requirements document. This document must be well-understood by all entities, especially quality assurance (QA), since it impacts regulatory assumptions and agreements. The next step is to design a system focused primarily on utility elimination, but also on integration. (As discussed, water, air, drains, and decontamination systems are all prime targets.) The result will be a rapidly deployed facility with a small footprint and low operation costs — something all pharmaceutical manufacturers are looking for.
Future installments in this series will explore how single-use systems can reduce footprints and enable closed systems to drive changes in HVAC (heating, ventilation, and air conditioning), utilities, and construction. Previous articles include:
- Implementing Single-Use At Bioprocessing’s Core
- An Introduction To Biopharmaceutical Facility Design & Layout
- Bioprocess Facility Design — Layout Rules and Configurations
- Are You Overlooking This Critical Component Of Bioprocess Facility Design?
About The Authors
Herman Bozenhardt has 40 years of experience in pharmaceutical, biotechnology, and medical device manufacturing, engineering, and compliance. He is a recognized expert in the area of aseptic filling facilities and systems and has extensive experience in the manufacture of therapeutic biologicals and vaccines. His current consulting work focuses on the areas of aseptic systems, biological manufacturing, and automation/computer systems. He has a B.S. in chemical engineering and an M.S. in system engineering, both from the Polytechnic Institute of Brooklyn.
Erich Bozenhardt is the lead for IPS-Integrated Project Services’ process group in Raleigh, NC.
He has 10 years of experience in the biotechnology and aseptic processing business and has led several biological manufacturing projects, including cell therapies, mammalian cell culture, and novel delivery systems. He has a B.S. in chemical engineering and an MBA, both from the University of Delaware.